Antibiotic resistance in animal pathogens
Introduction
The role of antibiotic therapy is to help the body destroy the bacteria without a detrimental effect on the host.
The introduction of penicillin and later other antibiotics meant that people had less to fear from serious diseases like the ‘black death’ or plague, sexually transmitted diseases and bacterial diseases leading to high infant mortality. Animals also benefited as they were able to remain healthy and food animals were able to use food more efficiently. Healthier animals also meant that humans were less likely to contract zoonotic infections either directly from their animals or by eating infected animal products.
However, our enthusiasm to treat these and any other diseases with antibiotics has encouraged antibiotic resistance development in microbes that reside in humans and animals. This is threatening our ability to effectively treat serious bacterial diseases. In fact, globally, antimicrobial resistance in serious bacterial pathogens is considered an emerging public health threat. Globally 700 000 humans die from antibiotic-resistant bacterial infections.
What is antibiotic resistance?
Antibiotic resistance is the capacity of bacteria to survive exposure to a defined concentration of an antibacterial substance.
A YouTube video introducing you to antibiotic resistance [9:09]:
Antimicrobial resistance is now identified by the WHO and WOAH as a serious global public health threat. The Australian government has a specific website, Antimicrobial Resistance, that provides all stakeholders, that is everyone, with information.
Is antibiotic resistance a recent phenomenon?
Bear in mind, antibiotic resistant microbes predate commercial antibiotics. Antibiotics were invented by bacteria, they use them to protect themselves from other microorganisms in complex communities such as the intestinal tract or organically rich warm environment. Many modern antibiotics such as streptomycin and penicillin have been developed from bacteriocins produced by bacteria and fungi respectively.
It is the use, especially overuse, of antibiotics that has increased the speed of antibiotic resistance development.
This is what Alexander Fleming the discoverer of penicillin wrote in 1945:
“the microbes are educated to resist penicillin and a host of penicillin-fast organisms is bred out … In such cases the thoughtless person playing with penicillin is morally responsible for the death of the man who finally succumbs to infection with the penicillin-resistant organism. I hope this evil can be averted.”
Research into the development of novel antibiotics has “hit a wall” with a reduced likelihood that completely novel, effective and safe antibiotics will be found or developed soon. This means that we have to manage our use of currently available antibiotics and investigate alternative ways in which to control bacterial infections.
Where do we find antibiotic resistant bacteria?
They are found in all environments: in the animal, human and plant microbiome as well as in the environment. Thus any control measures should address all possible sources of these bacteria.
Antibiotics labelled by the WHO as critically important to human health are those that are used to treat serious disease with often antibiotic resistant strains of bacteria. Those with resistance genes from non-human sources are included. The antimicrobials with the highest priority include the fluoroquinolones, macrolides, 3rd and 4th generation cephalosporins, vancomycin and colistin. Access to The WHO CIA list 2019.
The WHO has published a list of their priority antibiotic resistant bacteria shown in the Table below. The bacteria shown in bold are of greater concern to the veterinary community, not only because these resistant strains of bacteria cause disease in animals, but also because animals may play a role in transfer of these bacteria to people.
Priority | Antibiotic Resistant Bacterium | Significance and Role of Animals |
1 |
Carbapenem resistant
|
Carbapenems are not used in food animals and very rarely used for multidrug resistant infections in companion animals and reptiles. Reverse zoonosis risk, especially in animal hospitals |
Extended spectrum beta-lactamase (ESBL) producing Enterobacteriales |
Moderate to high risk as beta-lactam use is popular in animals. Zoonotic pathogens in this group. |
|
2 |
Vancomycin resistant
|
Low risk as glycopeptides are not used in animals. Animal-associated MRSA and MRSP possible, but they should be susceptible to vancomycin. Reverse zoonosis risk |
Clarithromycin resistant Helicobacter pylori |
Low risk as macrolide use in dogs and cats is rare. Low to moderate risk from commercial pigs |
|
Fluoroquinolone resistant
|
The use of fluoroquinolones in food animals is banned in Australia. Moderate risk from companion animals like dogs, cats, pocket pets and reptiles. |
|
Cephalosporin resistant Neisseria gonorrhoeae |
Low risk as this is a human-specific pathogen |
|
3 |
Penicillin resistant Streptococcus pneumoniae |
Low risk as this is predominantly a human pathogen. Reverse zoonosis risk |
Ampicillin resistant Haemophilus influenzae |
Low risk as this is a human-specific pathogen |
|
Fluoroquinolone resistant Shigella |
Low risk as this is a human-specific pathogen |
Learning Objectives
- Compare the differences between intrinsic Intrinsic Resistanceand acquired antibacterial resistance Acquired resistance by the provision of examples of each and discuss how this affects the selection of antibiotics.
- How do bacteria acquire resistance genes?
- Explain what the mutation prevention concentration (MPC) Mutation Prevention Concentration (MPC)of an antibiotic and why it is necessary to ensure that animal patients are treated using these concentrations.
- Explain some well known mechanisms that bacteria use to resist the effect of an antibacterials providing examples of each class of antibiotic.
- Define multidrug resistance (MDR)multidrug resistance and explain why this is a public and animal health problem.
- Name and discuss the principals of some tests that are used to detect antibiotic resistance
- Design a “Prudent Use of Antibiotics” program that is associated with knowledge on what drives antibiotic resistance and how it is disseminated.
Intrinsic resistance
This is an inherent characteristic of a particular species of microbe to a particular anti-infective agent. Genes coding for this form of resistance are usually within the microbe chromosome.
Therefore, it is important in most cases of life-threatening disease that you determine the cause. For example, Gram’s staining of cytology of infected exudates will assist you in selecting an appropriate anti-infective drug for your animal patient.
Learning Objective
Inactive bacteria – a special case
When bacteria are dividing rapidly and producing a lot of proteins and other substances, they are much more vulnerable to antibiotic attack. However, when they slow or stop non-essential metabolic services they become more resistant to those antibiotics that are dependent on active cell uptake or metabolically active bacteria be be effective.
When are bacteria less active?
- At the end of their log phase of growth when nutrients become limiting
- Bacterial spores
- In biofilms. Up to 80% of pathogenic bacteria that produce biofilms result in persistent infections. Read the next Section to find out why.
- Persistent bacteria. Bacteria not only persist in biofilms but also make use of cells i.e. brucellae in white blood cells within lymph nodes and immune-privileged sites i.e. Leptospira in the inner eye.
- Persister cell mutants. These are bacteria that survive in the presence of high concentrations antibiotics that should destroy them. An example is an Escherichia coli with a mutation in the ptsi gene that regulates the transmembrane transport of sugars.
- Stressed or threatened bacteria (see extrinsic resistance section for a more complete explanation). Bacteria that are threatened may alter their morphology to overcome the threat. For example, bacteria in the presence of cell wall active antibiotics that are sub-lethal may stop producing an outer membrane. These bacterial L-forms survive in a watery environment and once the inhibitor is depleted, it will again produce an outer membrane. Bacteria when subjected to a variety of stressors i.e. DNA or wall damage to antibiotics, immune destruction, low nutrients, extreme temperatures, grow but don’t produce the septa that are necessary for them to form new bacteria. This makes it difficult for protozoa in the environment and phagocytic cells to ingest them.
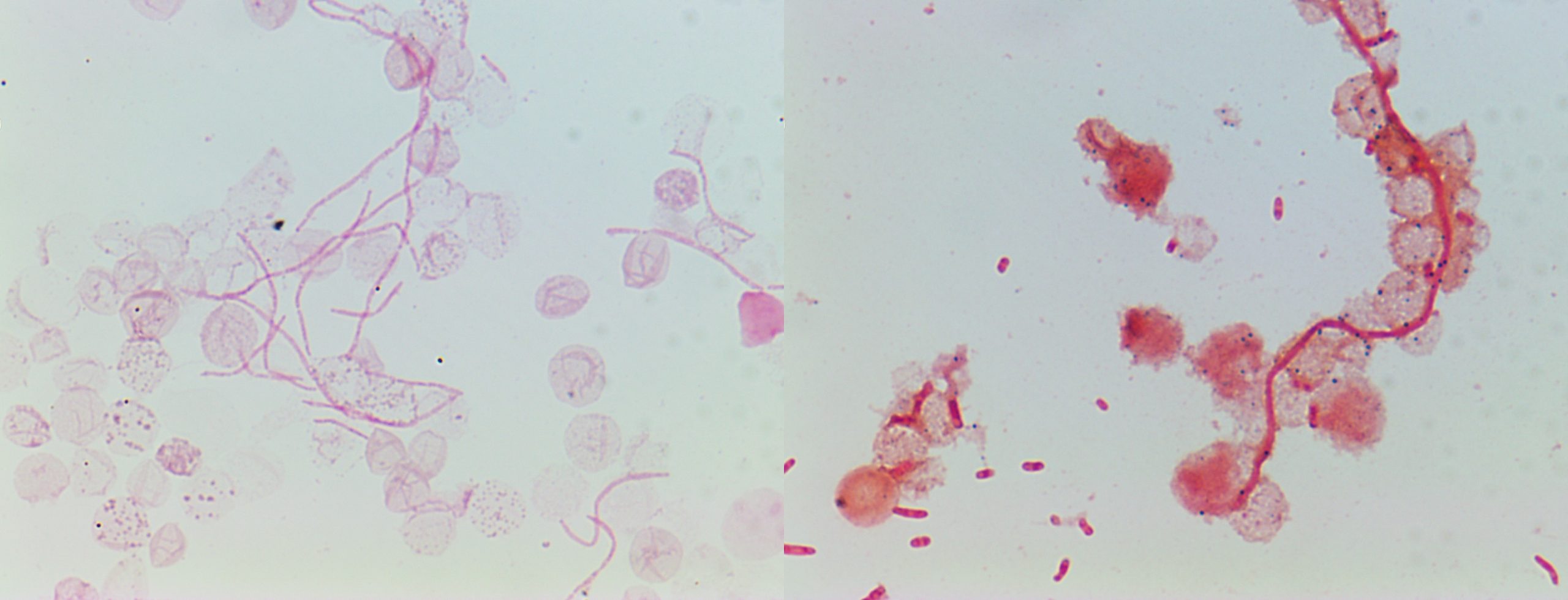
Biofilms and antibiotic resistance
Antibiotic-susceptible bacteria in biofilms are often protected from the action of antibiotics for the following reasons:
- Deep within a biofilm bacteria become inactive when they are deprived of oxygen and nutrients. This makes them less susceptible to the action of antibiotics that require an active transport mechanism to penetrate cells and to those antibiotics that negatively affect physiological processes involved in bacterial cell metabolism and growth. Examples of antibiotics requiring this are the beta-lactams, tetracyclines and fluoroquinolones.
- Some bacteria, whilst in a sessile state in biofilms, will produce antibacterial and antiphagocytic molecules i.e. S. aureus produces poly-γ-DL-glutamic acid (PGA) that makes it more resistant to neutrophil phagocytosis and binds to antimicrobial peptides (AMPs). AMPs are very small positively charged molecules that usually target bacterial membranes where they form porins or disrupt the cell membrane. Anti-AMP strategies by bacteria in biofilms can be overcome when combining AMPs with antibiotics.
- Some bacteria upregulate the production of sugars that bind to antibiotics, preventing their entry into bacterial cells. For example, ndvB gene up-regulated by P. aeruginosa in biofilms encodes the production of periplasmic cyclic glucan which binds aminoglycosides.
- Extracellular matrix can form an impenetrable barrier to antibiotics. For example, biofilms often accumulates organic acids which inactivate antibiotics that are inactive/insoluble in acids.
- Bacteria fixed in biofilms more easily acquire antibiotic resistance as their close proximity allows them to more easily exchange mobile resistance elements. Compared with their free-living bacterial relatives, they are more often subjected to sub-lethal concentrations of antibiotics and are more stressed. Thus they are more likely to mutate.
Acquired or extrinsic resistance
This is when bacteria acquire resistance to antibiotics either by the horizontal transfer of resistance genes or by gene mutations. This form of resistance can be promoted by the overuse or improper use of antibiotics. This type of resistance can only be detected in the laboratory, as simple knowledge of the cause is not predictive of Acquired resistance.
One of the first recorded and most publicized antibiotic resistanceAntimicrobial resistance was when strains of Staphylococcus aureus that were exposed to sub-killing concentrations of penicillinPenicillin developed resistance to beta-lactam drugs by the production of beta-lactamases. The blaZ gene encodes for beta-lactamases and is one of the reasons for methicillin resistance in Staphylococcus aureus (MRSA).
Learning Objective
How do bacteria acquire resistance genes?
Horizontal genetic material transfer
Bacteria can donate resistance gene sequences to other bacteria in the same ways as bacteria are able to change their genetic composition by 1. transduction (bacteriophage delivery), 2. conjugation (direct transfer of genes between related or unrelated bacteria by sexual pili) (most common) and 3. transformation (uptake of DNA from the environment when another bacterium lyses) (least common). Horizontal resistance gene transfer is very rapid especially when the more antibiotic resistant members of a bacterial population are able to predominate in the presence of antibiotic. Therefore it is important that treatments destroy all target bacteria within a population. Horizontal gene transfer can also occur between closely related species i.e. Salmonella can transfer resistance genes to E. coli.
Most resistance genes are acquired via horizontal transfer are found in transferable elements such as conjugative plasmids, insertional sequences and transposons. Integrons are often present in these mobile elements as they are assembly platforms that add genetic material to existing material and ensure their correct assembly and functionality. Mobile integron class 1Mobile class 1 integrons is the best known as they confer resistance to β-lactams, all aminoglycosides, chloramphenicol, trimethoprim, streptothricin, rifampin, erythromycin, phosphomycin, lincomycin and antiseptics of the quaternary-ammonium-compound family. They can also recruit novel resistance genes. More common in gram-negative bacteria, but also in some gram-positive bacteria. Studies focusing on antibiotic resistance in the environment often target this integron.
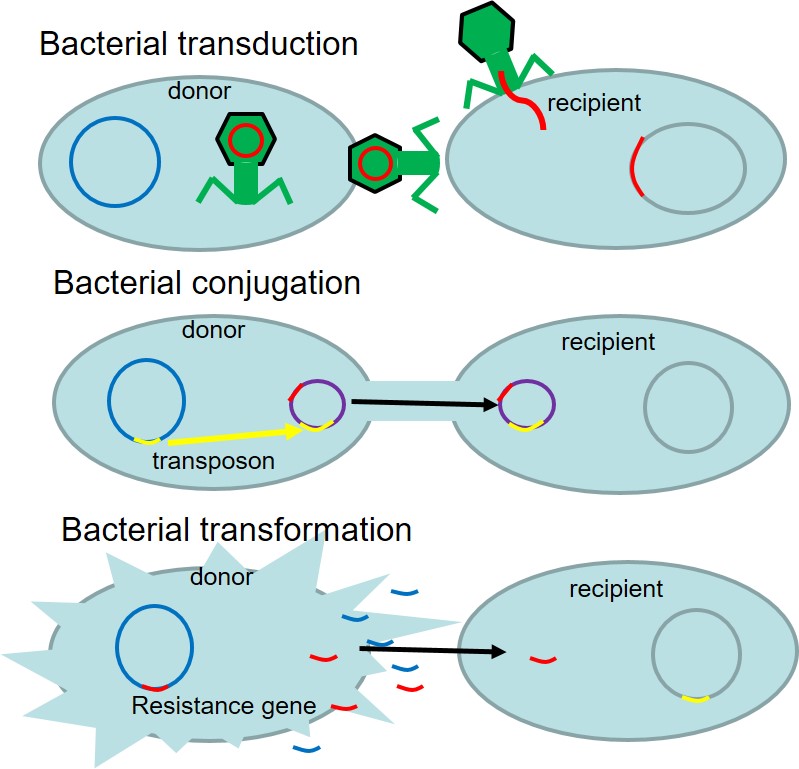
Gene mutations causing vertical transfer of genes
Learning Objectives
Explain what the mutation prevention concentration of an antibiotic is and why it is necessary to ensure that animal patients are treated using these concentrations.
Successful gene mutations are less common than the horizontal transfer of genes. However, it has been shown that in the presence of some antibiotics, especially those that damage bacterial DNA, bacteria will increase their mutation rate. This may in part be due to some bacteria, when threatened, will stop dividing and make use of their SOS gene repair mechanism where they bridge the damaged DNA with a Rec protein and make the repairs using low-fidelity DNA polymerases. The polymerases will “guess” which oligonucleotides to add to bridge the gap increasing the likelihood of mutations. For example, in the presence of quinolones or fluoroquinolones point mutations in the gyrA, gyrB and parC genes of gram-negative bacteria allow the manufacture of a new DNA topoisomerase that is resistant to the binding of quinolones, like nalidixic acid (single mutation in position 23 of the gyrA gene) and fluoroquinolones (multiple mutations in gyrA, gyrB and parC genes). Other antibiotics known to stimulate a similar SOS response are rifampicinrifampicin, fusidic acidfusidic acid and phosphomycinphosphomycin (fosfomycin). Note too that the generation time of bacteria is generally very rapid (10 to 20 minutes per generation) favouring the generation of successful mutants. Antibiotics must then be administered at bacteriocidal, not bacteriostatic, concentrations to avoid this. This is known as the Mutation Prevention Concentration (MPC) .
In the Table below you will note that when treating with the fluoroquinolones such as enrofloxacin, the quinolone, nalidixic acid becomes resistant earlier than the fluoroquinolone. This is because a single mutation in the DNA gyrases or Topoisomerasae II will prevent binding of the quinolones whereas the double mutation will also prevent finding of the fluorinated quinolones. This form of resistance is known as cross-resistance.
The SOS response of bacteria to the beta-lactams is different. When penicillin binding protein 3 is damaged, the cell will go into stasis and weather out antibiotic onslaught. The bacteria may also reduce outer cell membrane production resulting in filamentous forms or may even stop producing an outer cell membrane producing L-forms (see in intrinsic resistance section).
Stepwise point mutations in gram-negative bacteria induced by the fluoroquinolones.
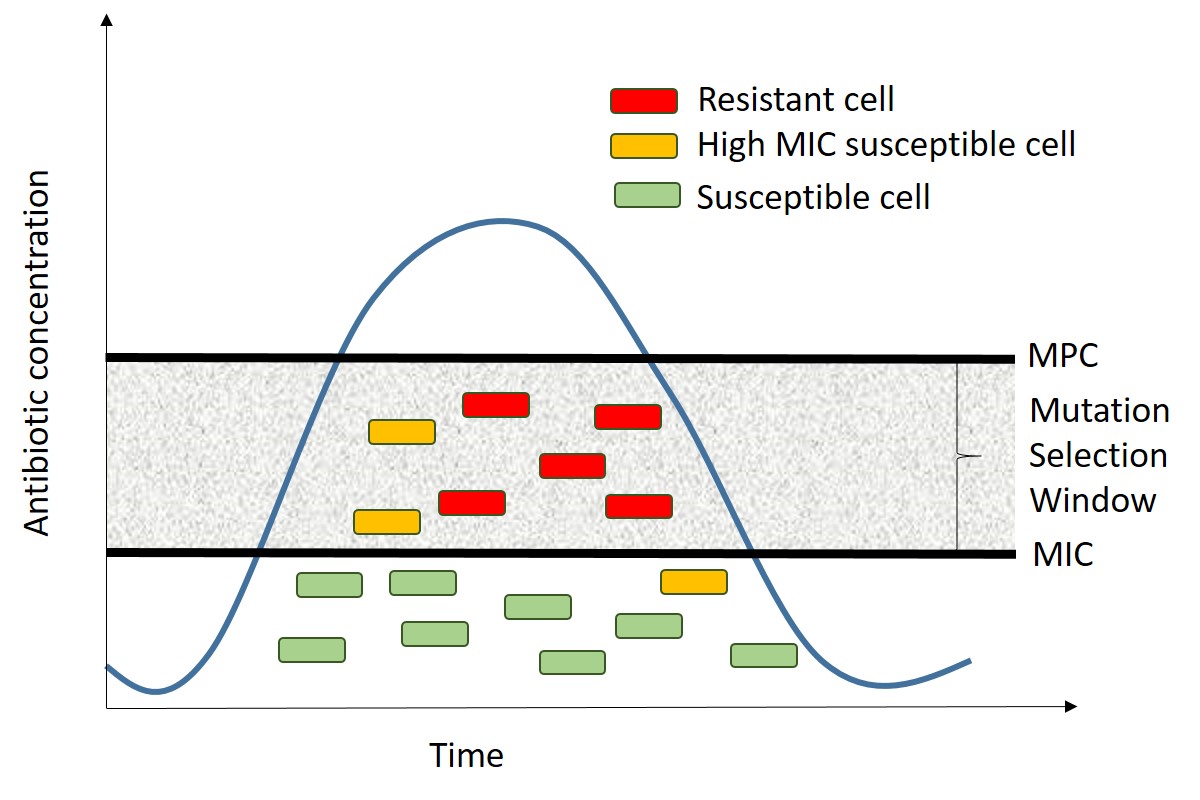
Heteroresistance
Heteroresistance is when a sub-population of a antimicrobial susceptible population shows resistance to an antibiotic.
Mechanisms bacteria use to resist antimicrobials
Bacteria make use of several anti-antimicrobial drug mechanisms and may use more than one of these to protect them against an antibiotic.
Learning Objective
Explain some well known mechanisms that bacteria use to resist the effect of an antibacterials providing examples of each class of antibiotic.
Broadly speaking, bacteria will make use of one or more of the following anti-antibiotic mechanisms:
- Destroy or modify antibiotic molecules by the use of enzymes i.e. beta-lactamases hydrolyse the beta-lactam ring of the penicillins and cephalosporins. Examples of these include blaZ encoded MRSA and extended-spectrum beta-lactamase (ESBL) production by the gram-negative coliforms. Chloramphenicol acetyltransferase produced by most bacterial species modifies chloramphenicol by attaching an acetyl group that stops the antibiotic from attaching to the 30S ribosome.
- Modify antibiotic target sites so that they are not recognised by the antibiotic. This confers a high level of resistance. mecA encoded MRSA produces altered penicillin binding proteins (PBP2a) = main reason for methicillin resistance in the staphylococci. Colistin resistance is thought to relate to lipopolysaccharide modification via changes in the mcrB gene and upregulation of PhoP/PhoQ. Some bacteria over-produce sulphonamide target sites, thus some target sites will not be blocked by sulphonamides allowing the bacterium to complete its folic acid cycle. Campylobacter tet(O) gene in the plasmid results in a protein that dislodges tetracycline from the 30S subunit. erm gene in S. aureus alters the macrolide/lincomycin/ clindamycin/streptogamin (MLS) binding site in the 30S ribosomal subunit. Staphylococcus, Campylobacter and Mycoplasma – the 23S ribosomal RNA is altered preventing attachment of macrolides. Note that this can also be step-wise with single mutation resulting in erythromycin resistance and multiple mutations in tylosin, tilmicosin and lincomycin resistance.
- Development of alternative metabolic pathways i.e. some bacteria alter PABA trimethoprim and the sulphonamides in the folic acid pathway. Excess folic acid provided by degranulating and dying cells in pus allows bacteria to survive even if their folic acid metabolic pathways are blocked by sulphonamides and trimethoprim.
- Manufacture specific or upregulate multidrug efflux pumps, preventing the accumulation of antibiotics within the bacterial cell. Gram-negative bacteria resistance to tetracycline is often by the presence of tetA or tetB genes which code for tetracycline specific membrane efflux pumps. The genes for multidrug systems i.e. AcrAB multidrug efflux pumps are usually located in the chromosome (intrinsic), but mutations or nucleotide transposition can result in up-regulation (increased activity) of these pumps. These tend to confer low-level resistance that are additive to other resistance mechanisms. These pumps work by the use of ATP, protons and ionic gradients.
- Modify membrane transport mechanisms i.e. Enterococcus species that are resistant to vancomycin have developed a thicker outer membrane that is rich in D-ALA residues which bind to vancomycin. Thus effectively blocking its entry into cells. E. coli OmpF porin allows hydrophilic antibiotics through the cell wall. Loss or alteration by decreasing their hydrophilic nature confers resistance. Loss of the specific membrane channel “OprD” prevents imipenem, a carbapenem, entry to Pseudomonas aeruginosa.
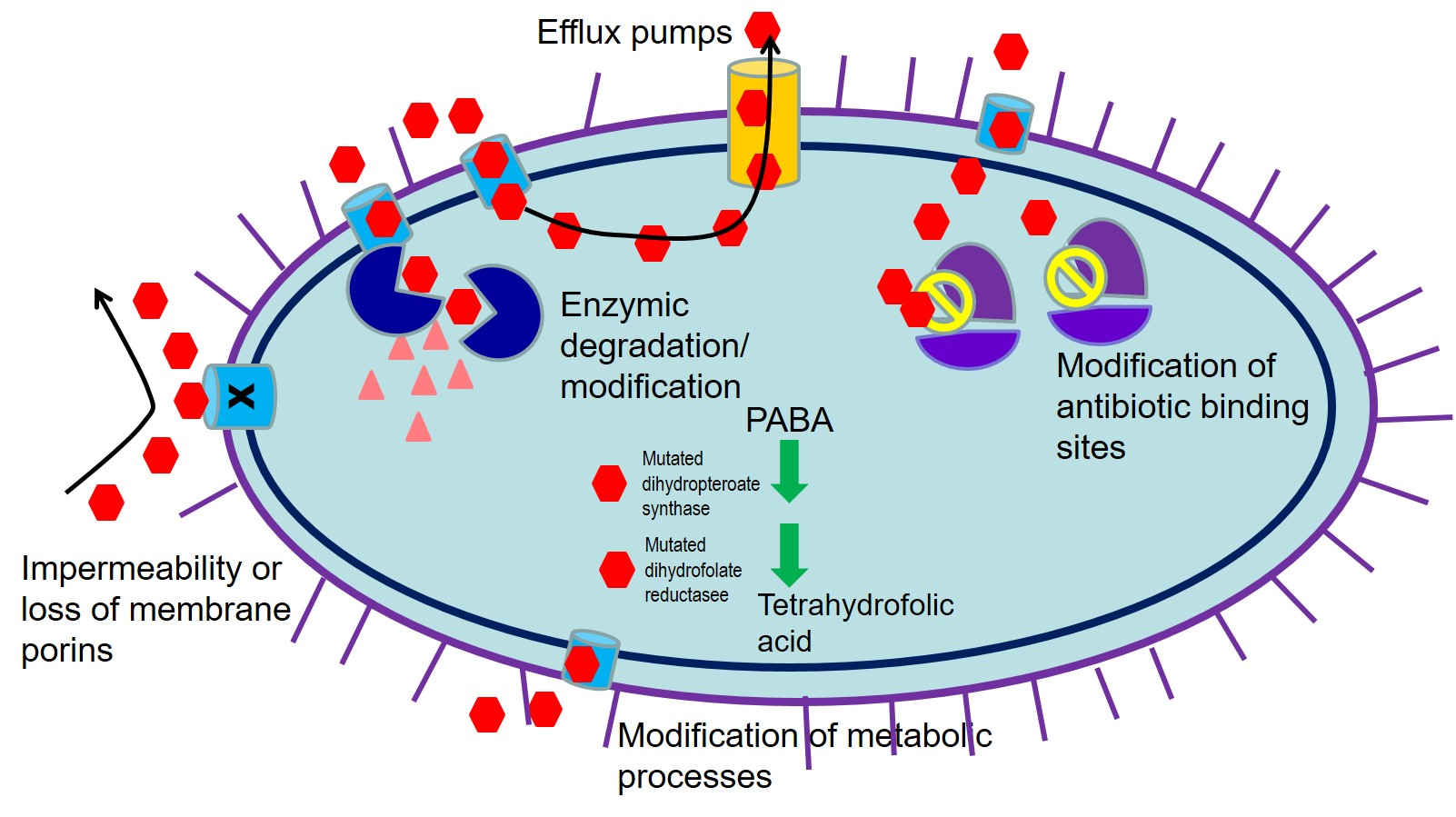
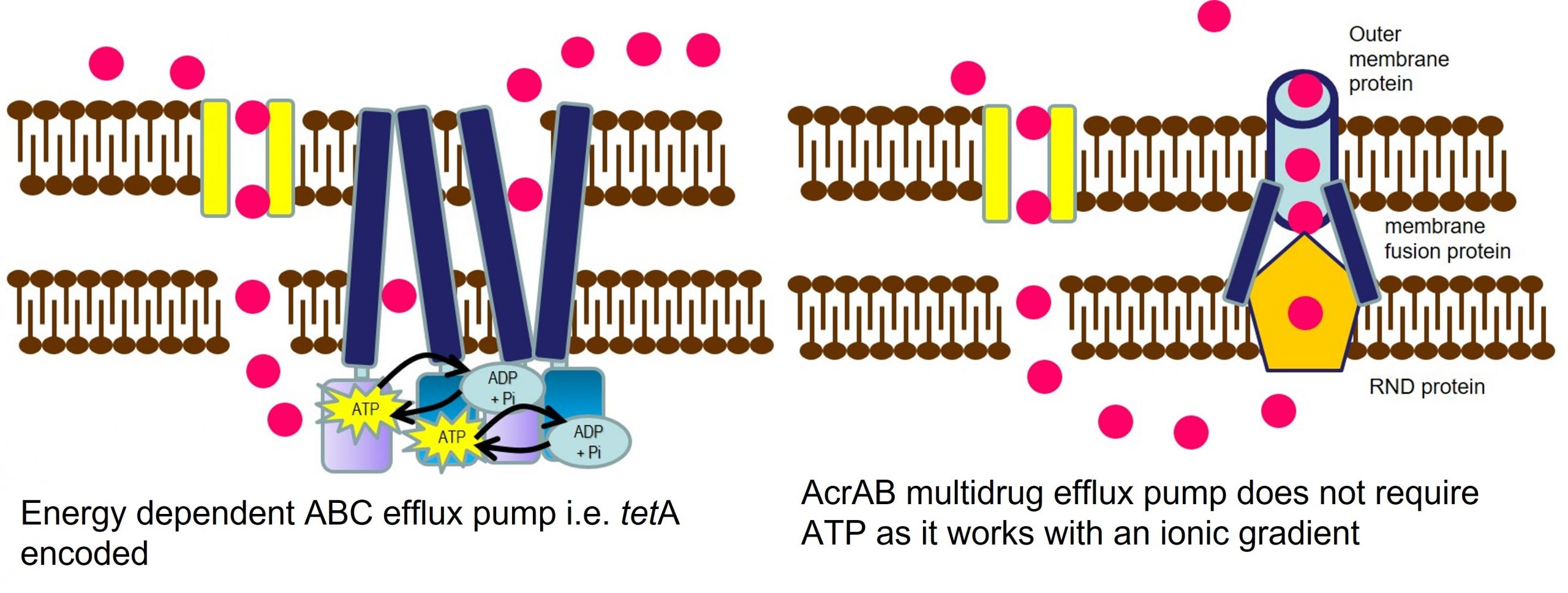
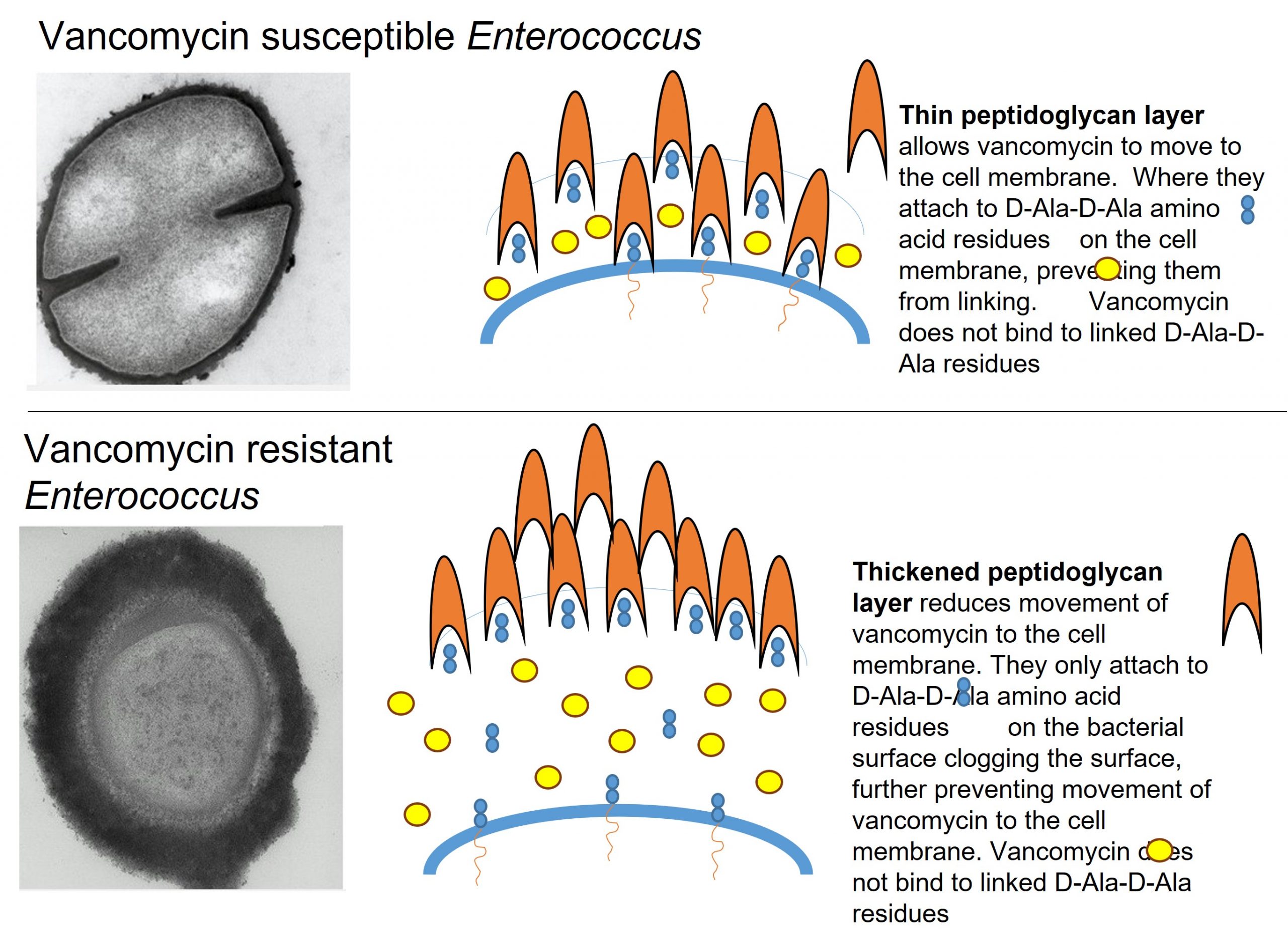
Drivers of antimicrobial resistance
Even before the extensive use of antibiotics, bacteria had already developed means of surviving antibacterial onslaughts. However, these bacteria were generally non-pathogenic and in low numbers in their normal habitat. With the extensive medical, veterinary and agricultural use of antibiotics this pattern has changed. Increasingly certain pathogenic bacteria have developed resistance to many commonly used antibiotics meaning that the treatment of life-threatening infections has to be done with expensive and potentially toxic antibiotics. In some instances, even those treatments are not working.
Whilst less publicised in animals, we have a similar situation in companion animals in Australia and in all domesticated or farmed animals in countries that don’t regulate the use of antibiotics.
The only way to tackle this problem is to take a “One Health” approach. This means that all Human, Animal and Agricultural Sectors using antibiotics should understand how each one drives resistance and their role in the environmental dissemination of antibiotics and resistant bacteria. This will allow each sector to institute measures that restrict the dissemination of resistant bacteria.
Learning Objectives
Define multidrug resistance and explain why this is a public and animal problem.
In situations where bacterial populations may be exposed to more than one antibiotic category or may come into contact with and acquire resistance genes from bacteria already resistant to some antibiotics, a situation where bacteria are resistant to all used antibiotics may occur. This has happened in hospitals, intensive livestock operations as well as in individual animals where treatment has not been effective.
A bacterium that has acquired resistance to at least one antibiotic in 3 or more antibiotic categories is considered to be multidrug resistant (MDR). A bacterium that is resistant to at least one antibiotic in most antibiotic categories except for one or two is known as extensively drug resistant (XDT). Note that they need only be resistant to one drug in a category. A bacterium that is resistant to all antibiotics and all antibiotic categories is known as pan drug resistant (PDR).
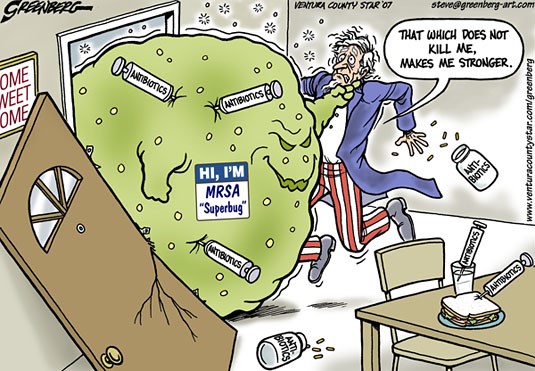
The case of Salmonella Typhimurium DT104 (DT104)
First resistant strains of DT104 were detected in people in Thailand in the 1970s. A new resistant strain of DT104 emerged in Europe in the mid to late 70s, it spread extensively in Europe and USA and the rest of the world. It was detected in the UK in wild birds in early 1980s, spread to cattle and then humans by 1989. Also spread to poultry, pigs and sheep over next 5 years. The highest number of annual cases in people is in Europe and North America. Whilst DT104 was introduced into Australia, it never gained a foothold. Most cases nowadays are in people returning from visits to SE Asia. Transglobal movement of DT104 is likely via foods. By 1984 it carried resistant genes to A (ampicillin), C (chloramphenicol), S (streptomycin), Su (sulphonamides) and T (tetracycline). By 1997, additional resistance to trimethoprim and decreased susceptibility to the fluoroquinolones (ciprofloxacin). Resistance was found to be chromosomal within Mobile class 1 integronszones. This bacterium is what made the world realize the One Health nature of antibiotic resistance.
This is a case of co-resistance. Co-resistance occurs when there is resistance to more than one antibiotic category where the encoding genes are transferred in the same mobile element.
Mechanisms driving antimicrobial resistance
There is no doubt that antibiotic resistance development and the global burden is hastened by excessive use of antibiotics.
Below are 3 proposed mechanisms:
- Resistance pressure (vertical transmission). A microbial population is never homologous, even if a pathogen has been derived from the same clone. Thus the exposure of this population to sub-lethal concentrations of an antibiotic may lead to the destruction of the very susceptible bacteria but not those tending towards resistance. This will allow those bacteria tending towards resistance to divide and predominate in the population. In individuals this then leads to apparent treatment failure. In an individual this can happen in 5 to 10 bacterial generations. So fast! A list of drivers is described in the next section. Note that this pressure can be reversed by not using the culprit antibiotic. However, a small proportion of the resistant population will remain. Another route is to ensure that all the pathogens are killed before this happens.
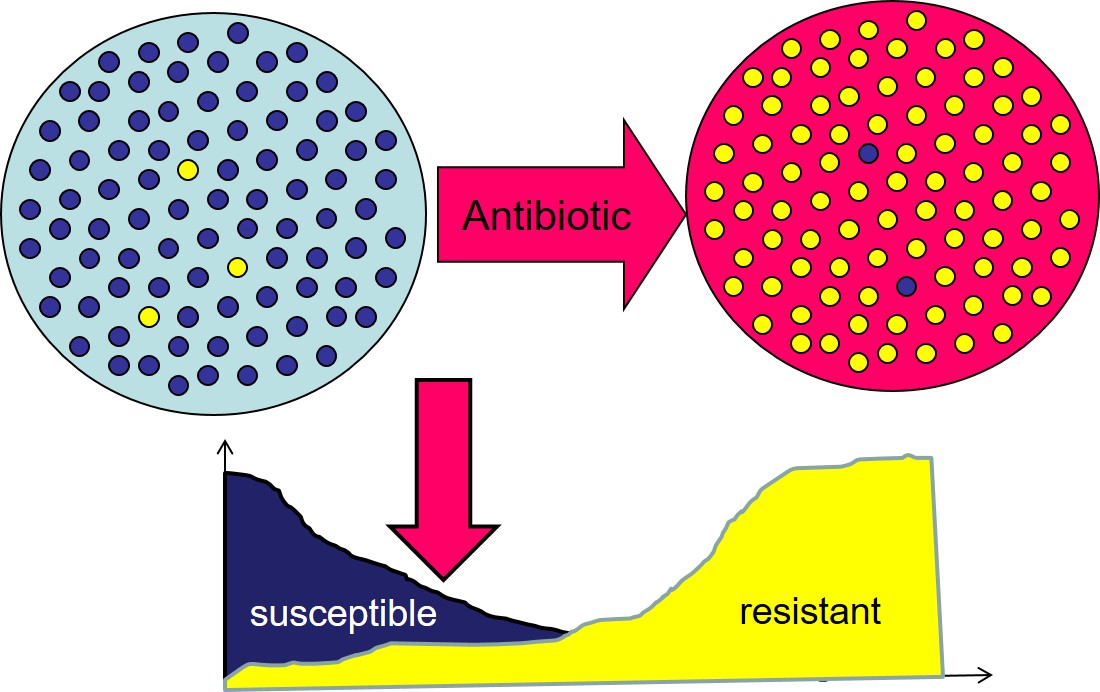
2. Horizontal transfer of resistance genes. When resistant bacterial populations are allowed to predominant in the presence of antibiotics in mixed bacterial populations i.e. in the gastrointestinal tract they can transfer resistance genes horizontally via mobile plasmids or transposons to related susceptible bacteria within the same environment. This occurrence is common in the Enterobacteriales. Since some of these mobile elements may have resistance genes to a number of different antibiotic categories a pattern of multidrug resistance may be observed, even if not all the antibiotics were used. (See section above on co-resistance).
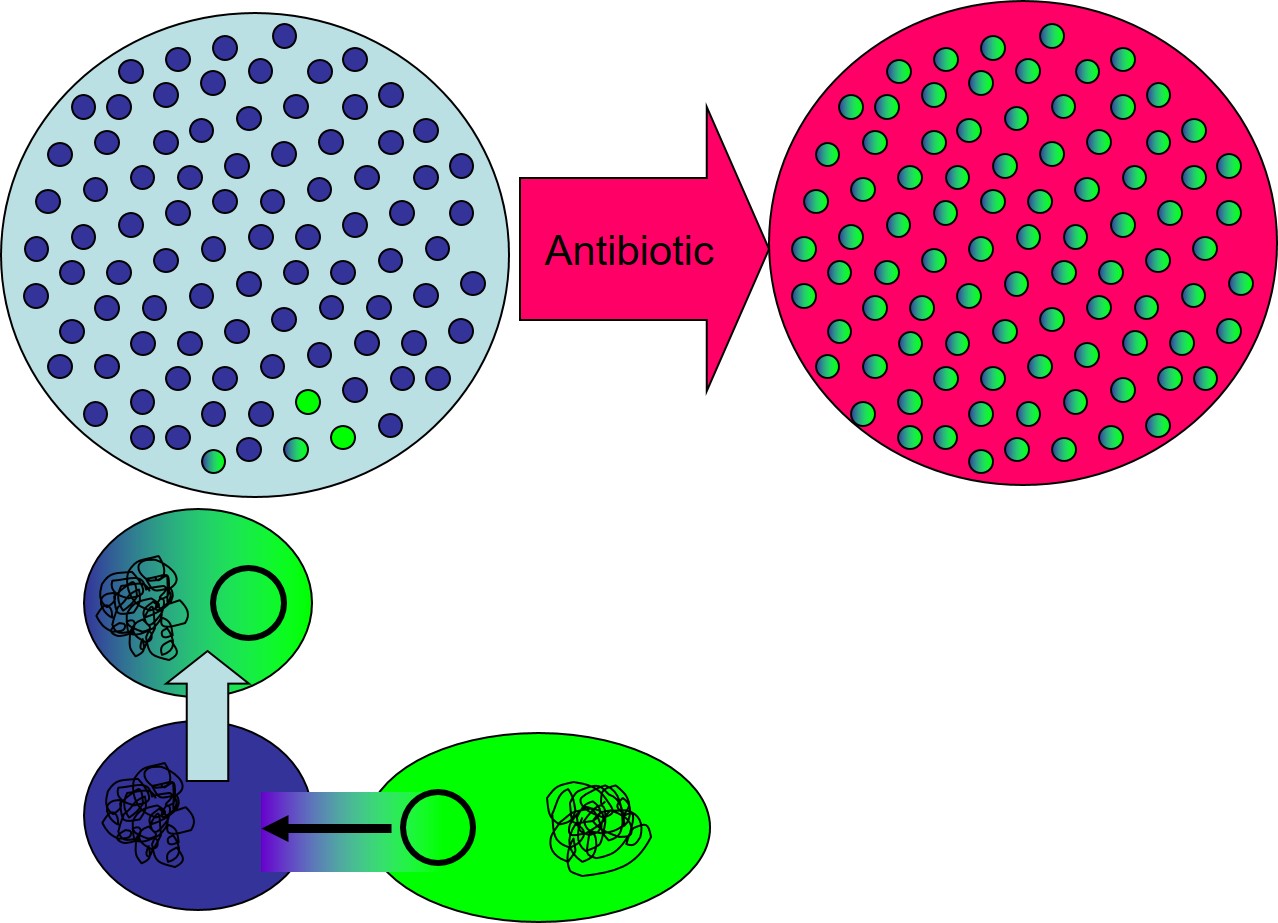
3. Mutagenesis. Whilst most mutations are destructive to bacteria, some will not be. Mutations that allow a tiny proportion of a microbial population to survive will eventually predominate as the susceptible bacteria are killed under the selection pressure of antibiotics. In fact, an increased rate of mutation may occur in damaged bacteria as part of their SOS response (See extrinsic resistance)
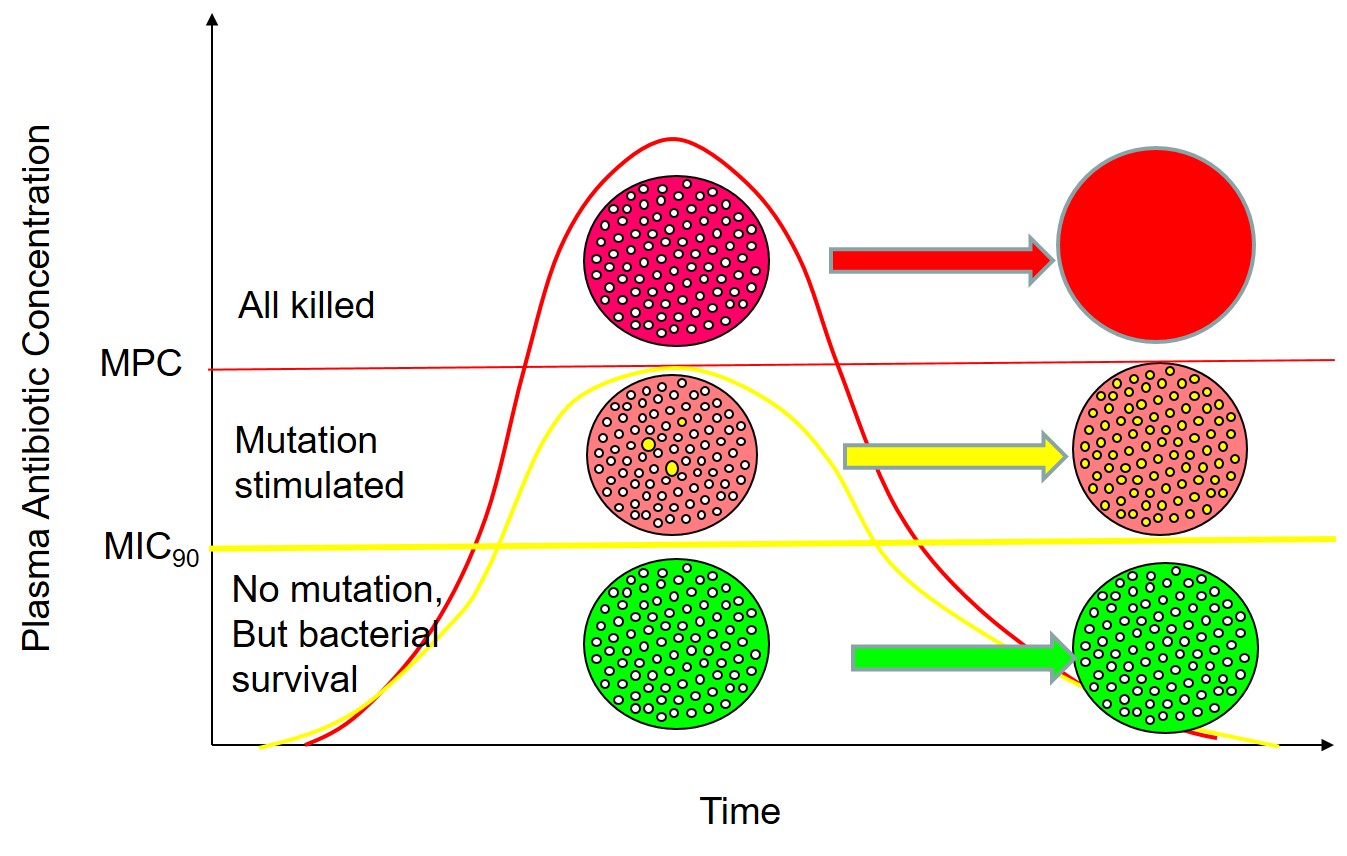
4. Replacement with resistant bacteria
Treatment of the bacterial infection without lesion repair and reduction in inflammation in tissues can lead to a secondary infection with more antibiotic resistant bacteria. A good example is otitis externa. A dog may be suffering from atopy or allergies and is constantly scratching his ear. This allows pathogenic staphylococci to infect the ear. It is successfully treated with antibiotics but the allergy or atopy is not fully controlled. The ear is still inflamed but only bacteria resistant to the antibiotics will survive. Thus, excessive growth of the less pathogenic, but multidrug resistant bacterium, Pseudomonas aeruginosa and the yeast Malassezia is facilitated. In this case, the lesion will initially appear to have improved, then relapse and become even worse.
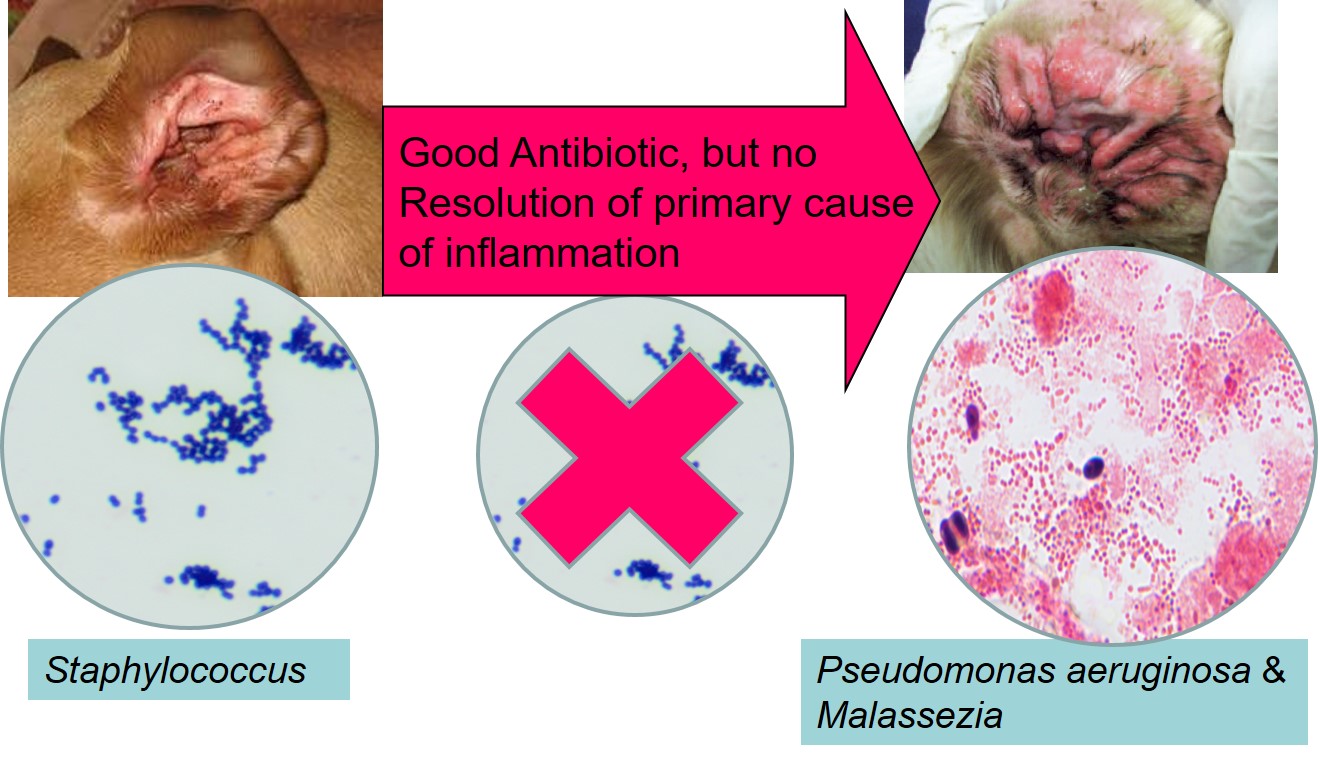
What practices or behaviours encourage antibiotic resistance pressure?
-
Inappropriate therapeutic use of antibiotics
This is arguably the most important reason for increasing resistance pressure.
When does this happen:
- There is no evidence that the infection is caused by bacteria i.e. treating uncomplicated viral disease with antibiotics or treating diarrhoea due to a bacterial toxin with antibiotics.
- Using the incorrect antibiotic i.e. treating a gram-positive infection with a gram-negative active antibiotic. As culture results can take a few days, knowledge of the disease and direct cytology of lesions can guide you. It is important to test for resistance and change the treatment if the antibiotic is shown to be ineffective.
- Using too low a dose or not following dose recommendations i.e. owners finding it difficult to administer drugs to a cat. Some people will stop administering treatments when the pet is well again – consider administering long-action, injectable antibiotics i.e. Convenia. (But be careful – see below)
- Using antibiotics long-term without checking for a clinical or microbiological response.
- Treating minor infections with antibiotics.
- Using a broad-spectrum antibiotic when a narrow spectrum will do – causes harm to the normal and protective microflora and may encourage resistance in normal microflora as well as a dysbiosis – as the normal microflora is protective. (Normal microflora can act as opportunistic pathogens and could be a source of mobile antibiotic resistance elements).
- Using systemic therapy when local or topical therapy would be more effective i.e. some skin, ear and eye infections. Note that much higher concentrations of antibiotics can usually be attained in topical and other local therapies than systemic therapy.
- Using long-acting antibiotics against bacteria with high minimum inhibitory concentration (MIC)[/pb_glossary]s. Convenia cefovecin (Convenia)is long-acting as it is slowly released from its plasma-bonded state. However, it is shorter acting against the gram-negative bacterium than the gram-positive bacteria and generally more shorter acting in dogs than cats. It also is dependent on normal plasma protein levels. Thus sub-therapeutic tissue concentrations of this cephalosporin may occur.
2. Use of antibiotics for mass medication (therapeutic and non-therapeutic use)
Mass in-food or in-water medication in food animals is a common practice on many intensive farms. When there is an outbreak of a diagnosed contagious disease, this type of treatment is practical and will reduce the disease burden. When used to treat a group of sick animals it is therapeutic and when used to prevent spread of the contagious bacterium to a susceptible group of animals it is known as metaphylaxis. It is also used in anticipation of disease or prophylactically i.e. in farms endemic for enzootic pneumonia in swine when there is an age related peak in disease. Mass medication is often effective, but not without risk of antibiotic resistance development. Some of the reasons for this include:
- Given to large numbers of animals for prolonged periods increasing the opportunity for resistance development and dissemination.
- Variable concentration intake of the medicated feed or water. Sick animals don’t eat well so may ingest sub-therapeutic dosages of antibiotics. Animals with a fever response will drink more than those that don’t have one. Certain antibiotics may make the feed or water less palatable. Other factors include poor mixing of medications in the feed, precipitation of medications in water and inactivation of the antibiotics.
- Incorrect antibiotic. No knowledge of the current antibiotic resistance pattern of the potentiated pathogens. It may also lead to broad-spectrum antibiotics being selected. This can be overcome with the use of targeted disease surveillance.
- If not properly managed these medications may enter the groundwater and disseminate. This risk is greatest in aquaculture and crop agriculture. It is theorised that the mobile resistance elements of the multi-drug resistant Salmonella DT104 originated from antibiotic use in aquaculture. Evidence for this is the presence of the florfenicol resistance and tetracycline class G genes in the resistance cassette that have both been traced to Vibrio species from farmed marine fish in the Far East.
3. Use of antibiotics as growth promoters (non-therapeutic antibiotics)
Antibacterial growth promoters are believed to reduce the number of bacteria colonising the intestinal tract. This leads to more food being available for intestinal absorption and a thinner intestinal mucosa allowing easier uptake of nutrients by the intestinal tract. These antibiotics tend to be narrow-spectrum, are not absorbed from the intestinal tract and target gram-positive bacteria, spirochaetes and some gram-negative bacteria, but not the Enterobacteriales. However, they are administered orally at sub-killing doses for prolonged periods, encouraging bacterial resistance of intestinal microflora. In Australia, the use of antibiotics as growth promoters is restricted. The EU and USA (2017) have banned the use of antibiotic growth promoters in food animals. This, however, is not true for many countries. It must be noted that countries that don’t use growth promoters have higher incidences of gastrointestinal disease in livestock, which may lead to a high use of therapeutic antibiotics. An example is after the banning of antibiotic growth promoters in the feed, the pig industry in Europe had an increased incidence of swine dysentery in commercial grower pigs. This increased use of therapeutics declined as animal husbandry practices improved.
Quite a lot of work has been done to look for associations between multi-drug resistant infections in people and their food sources. Work in the late 1990s showed that vancomycin resistant Enterococcus faecium infection in people in Norway was associated with the same ribotype of this species in pig intestines. The vancomycin resistance occurred due to cross resistance from the growth promoter avoparcin, a glycopeptide like vancomycin, that was fed to the pigs. From 1995 – 2000, avoparcin was banned in many countries and global production of the drug ceased. So it is no longer available, even in countries that don’t ban its use. Note that Australia does not allow gentamicin, colistin, fluoroquinolones and 4th generation cephalosporins to be used in food animals.
Dissemination of antimicrobial resistance
Active antimicrobial residues and antimicrobial resistant bacteria can disseminate widely in the environment and to all living creatures. Environmental and commensal bacteria can also transfer mobile genetic elements that encode for antibiotic resistance to pathogenic bacteria. This means that no living creature is free from the effects of antimicrobial resistance. It is truly a global issue and of great interest to public health.
It must be emphasized that most of the negative effects of antimicrobial resistance in people is from the overuse of antibiotics in people. Globally, 90% of infectious agent related deaths in people are caused by tuberculosis, malaria, pneumonia, HIV/AIDS, diarrhoea and measles. Of these, antibiotic therapy in animals can play a role in deaths associated with pneumonia and diarrhoea. Food-borne infections caused by Campylobacter, Salmonella, Shigatoxin producing Escherichia coli and Yersinia are more serious when the cause is antibiotic-resistant bacteria. In the USA, outbreaks of fluoroquinolone resistant Campylobacter jejuni enteritis in people, with chicken meat and eggs being the source, led to the banning of the use of enrofloxacin in food animals.
Close contact with other individuals also allows antibiotic resistant bacteria to be transferred between individuals. It has been shown that people can transfer their resistant bacteria to the animals they are in contract with i.e. pet owners to their pets and vice versa and farm workers to the animals they work with and vice versa. One of the major concerns is the transfer of resistant bacteria within a hospital situation either directly from hospital staff to animals or indirectly via inanimate objects. In the literature there are numerous examples of MRSAmethicillin resistance Staphylococcus aureus nosocomial infections both in equine and small companion animal hospitals. Classically in animal hospitals this has shown to be hair clipper blades, but any other objects may play a role. A high level of cleanliness is necessary when handling indwelling catheters as they can allow biofilms of antibiotic resistant bacteria to creep up the catheter into the body leading to sepsis in susceptible individuals. Thus particular care should be taken when working with immune-suppressed patients.
Groundwater has been shown to be one of the most important disseminators of antibiotics and antibiotic resistant microbes. The level of dissemination is proportional to the usage of antibiotics in humans, animals and plants. All antibiotics that are excreted in active form either in the urine or faeces may find their way into groundwater and contaminate the environment. Presence of active antibiotics at low concentrations will stimulate the development of antibiotic resistance in the microflora of the soil, plants, animals and humans that are in contact with these antibiotics (See drivers of antibiotic resistance). Currently antibiotics exceeding 300x the limit are found in the Nairobi River in Kenya (sulphamethoxyzole), and rivers in Nigeria, Ghana, Bangladesh and Pakistan. Countries with high levels of AMR are those where over-the-counter and black market antibiotic sales occur i.e. India and the aforementioned countries. In some European countries, like Italy antimicrobial use increased in hospitals due to COVID-19 infections. Recent studies in fish farms in SE Asia show that there are a high level of antibiotic resistance in Aeromonas and Vibrio species – even to antibiotic classes not used in animals i.e. aminoglycosides.
Resistance mechanisms to some important antibiotics
In this section, I will only highlight those resistances that have a global impact on antimicrobial resistance in public and animal health.
Learning Objectives
Explain some well known mechanisms that bacteria use to resist the effect of antibacterials providing examples of each class of antibiotic.
Beta-lactam drugs
Use of members of this class of drugs are wide as they are relatively safe and dependent on the class, they treat both gram-positive and gram-negative bacterial infections. Earlier generation beta-lactam drugs are usually 1st tier and can be used for empirical therapy.
Two types of acquired resistance to them are of concern:
- Methicillin-resistant staphylococci (MRS)
- Extended beta-lactamase (ESBL) resistance by members of the Enterobacteriales
1. Methicillin-resistant Staphylococcus (MRS) infections
Methicillin-resistant Staphylococcus (MRS) are resistant to all currently available β-lactam antimicrobials and carbapenems.
Specific staphylococci:
- Methicillin resistant Staphylococcus aureus (MRSA) = Spillover from human hospitals via pet owners or originate from animals i.e. mastitis milk and pigs
- Methicillin-resistant Staphylococcus pseudintermedius (MRSP) = ZOONOTIC potential; Nosocomial in dog veterinary practices
MRS species have acquired a mobile genetic element known as the staphylococcal cassette chromosome (SCC). The SCC carries the mecA gene that encodes for the production of an altered penicillin-binding protein (PBP2a). This protein is able to manufacture the bacterial outer membrane but does not allow binding of β-lactam antimicrobials to it. Note that these bacteria are also resistant to potentiated penicillins, like amoxycillin plus clavulanic acid.
Another resistant gene known as the blaZ gene found in the chromosome or plasmid encodes for beta-lactamase production. In animals, especially cattle, it is more common than mecA. In fact, about 60% of staphylocicci isolated from dogs have this gene.
The SCC also contains insertion sequences that allow incorporation of additional antimicrobial resistance markers. These insertion sequences are why many MRS are resistant to non-β-lactam antimicrobials. MRS can be resistant to erythromycin, clindamycin, fluoroquinolones and tetracyclines. Some strains are even resistant to vancomycin, the so-called VRSA (Vancomycin-Resistant Staphylococcus aureus) a treatment for MRSA in people. Thus, reserve vancomycin for human use only.
Zoonotic MRSA spread by contact between humans and animals. For example: in Europe MRSA strain CC398 is the predominant strain in pigs where it is a commensal. It is increasingly being detected in people as it can also evade the human immune system. This methicillin-resistant strain is also resistant to tetracyclines and heavy metals.
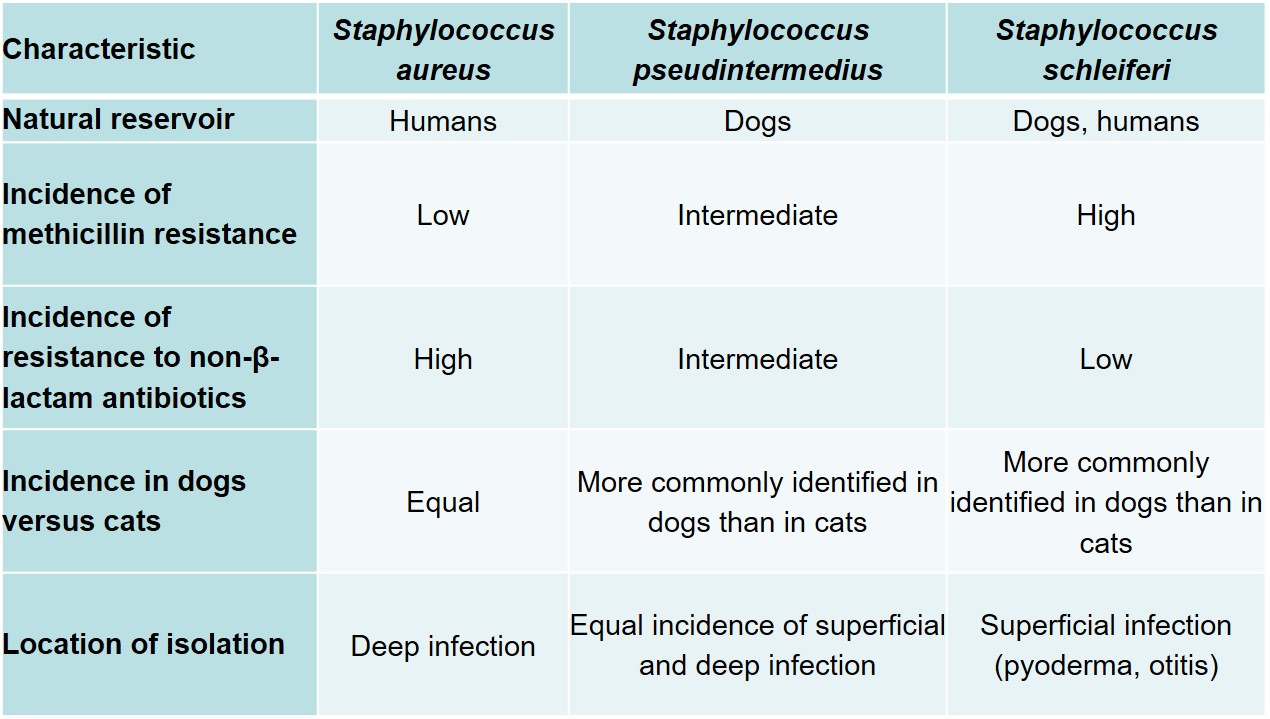
2. Extended-Spectrum beta-lactamase (ESBL)Extended-spectrum beta-lactamases (ES – producing gram-negative bacteria
The ability of enteric gram-negative bacteria to produce extended spectrum beta-lactamase (ESBL) is the most common form of resistance. In fact the presence of ESBL producing Escherichia coli can be used as an indicator of whether measures taken to reduce antimicrobial resistance are working.
Several plasmid-associated genes encode for the production of beta-lactamases in gram-negative bacteria. Some are more narrow-spectrum and others are very broad-spectrum. ESBLs are plasmid-associated beta lactamases that are capable of hydrolyzing penicillins, many narrow spectrum cephalosporins, many extended-spectrum cephalosporins and oxyimino-cephalosporins (cefotaxime, ceftazidime). An example of these are the AmpC-lactamase cephalinosporinases. Recently AmpC producing Enterobacteiles ave developed mutations in their membrane proin genes that make them less permeable to antibiotics. Thus their spectrum of antibiotics they are resistant to have increased to include the carbapenems (impenum and meropenem). Beta-lactamase inhibitors (e.g. clavulanic acid) generally inhibit ESBL producing strains. ESBL producing isolates are most common in Klebsiella spp., predominantly Klebsiella pneumoniae, E. coli and Proteus spp..
A group of extended spectrum beta-lactamases known as the carbapenemases are increasingly being recorded in human health. A concerning example of a carbapenemase is NDM-1 (New Delhi metallo-beta-lactamase) containing bacteria like some strains of Klebsiella pneumoniae, Escherichia coli, Acinetobacter spp. and Pseudomonas aeruginosa. These bacteria are resistant to all the beta-lactam antibiotics and the carbapenems. They are not resistant to the monobactams (aztreonam). Unluckily, a resistance gene to the monobactams is on the same gene cassette as NDM-1. Additionally, fluoroquinolone and aminoglycoside resistance is often on the same gene cassette. The carbapemens are hardly ever used in animals as they are banned in food animals and can only used to treat individual companion animals infected with multidrug resistant bacteria. Thus animals are generally not the source of this very high level of antibiotic resistant bacteria. However, companion animals live in close association with people – thus they are still at risk of becoming colonised or infected with carbaenemase producing bacteria.
3. Colistin (polymixin E) encoded in the plasmid transmissible genes mcr-1 and mcr-2 of the Enterobacteriales
The genes mcr-1 and mcr-2 are more common in Salmonella enterica, Klebsiella pneumoniae and Escherichia coli. Colistin is a locally active polypeptide antibiotic that has been used for a long time to treat scours in animals (not in Australia). However, with the advent of antibiotic resistance of human bacterial pathogens to other more commonly used antibiotics, it is increasingly being used parenterally, as a last resort to treat humans for life-threatening gram-negative infections that are resistant to carbapenems. Sources of colistin resistant bacteria have been found in animal products such as poultry and pig meat (the last recorded case in people originated from chicken that had been imported into Denmark from China). Plasmid-transmitted resistance to colistin has not been recorded in Australia. This is the first time plasmids (genetic mobile element) have been found to transfer colistin resistance. Previously reported resistance was chromosomal.
Cross-resistance is also likely to polymixin B, a local antibiotic used to treat ear, eye and skin infections. This antibiotic is used for topical therapy in animals in Australia.
Resistance of gram-negative bacteria to the polypeptides is due to alterations in proteins that regulate the manufacture of Lipid A (endotoxin) resulting in modifications of Lipid A so that polymixin has less affinity for it. Polymixin disrupts the outer membrane of gram-negative bacteria by displacing Ca2+ and Mg2+ ions making the outer membrane less stable. The drug also attaches to Lipid A in the bloodstream reducing the toxicity of Lipid A.
4. Macrolide resistance in mycoplasmas
Mycoplasmas are highly labile, require a very complex media and grow relatively slowly. Therefore antibiotic resistance in this group of microorganisms has been difficult to determine. Antibiotic classes that are used to treat this group of bacteria are the macrolides, tetracyclines and fluoroquinolones. In livestock, macrolides are preferred for the treatment of the respiratory mycoplasmas.
Recent, evidence shows that for some common mycoplasmal pathogens i.e. human M. pneumoniae and bovine M. bovis resistance to macrolides can be high in some populations.
5. Antifungal resistance
Antifungals in medicine and veterinary science are rarely used, thus, antifungal resistance has received a lot less attention than antibacterial resistance. However, increasingly fungi causing mycoses in humans and animals are shown to be unusually resistant to commonly used antifungals. Examples are multidrug resistant Candida auris in people that causes ear and bloodstream infections, azole resistant Aspergillus fumigatus, and multi-drug resistance in the fungi causing dermatophytosis. Thoughts are, that widespread treatment of crops with antifungals is to blame. Worldwide 99% of sales of antifungals are for crop treatment and the triazoles can remain unchanged in soils for several months.
Why test for antibiotic resistance?
Whilst treating empirically is the most practical way to respond to life-threatening infections. This decision should be informed i.e. evidence-based
1. What are the most likely aetiologies the clinical signs and animal/herd history are indicative of = differential diagnosis
2. What aetiologies have the rapid tests indicated? Where and when possible make use of cytology of lesions or infected fluids. This is a very useful tool that will assist you in deciding whether it is an infection and what type of pathogen is involved. Any rapid lateral flow assays that can be used. Thus it will inform your choice of therapy.
When should you consider a laboratory diagnosis?
- When animals have not responded or are responding inadequately to empirical therapy
- When the disease process is chronic
- When you suspect a nosocomial infection
- When the suspect cause requires 2nd or 3rd tier antibiotics
- When dealing with diseases where antimicrobial resistance is common
Learning Objectives
Name and discuss the principles of some tests that are used to detect antibiotic resistance
What tests are available?
- Bacterial culture and antibiotic susceptibility testing. Isolation of the causative bacteria or fungi by culturing is still the cheapest and most accurate way to determine a bacterial cause and phenotypic resistance patternphenotypic resistance pattern of the agent.
- Molecular detection of resistance genes. This is done where culture is difficult or too slow i.e. for mycobacteria. As this technology is becoming modified for in-practice use, it may become the primary way of diagnosis.
Testing for phenotypic antibiotic resistancephenotypic resistance pattern
They test whether the growth of a disease-causing bacteria is inhibited by specific concentrations of antibiotics.
Types of test:
- The disk diffusion = qualitative
- Broth/agar dilution test (MIC) = quantitative
- Agar gradient test (E-test)
- PCR to detect specific resistance genes
- Lateral flow assay/ rapid antigen test to detect specific resistances
- Selective culture to detect specific resistances
The disk diffusion test
The most common test used for routine testing is the disk diffusion test.
In this test, a filter paper disk infused with a known concentration of a particular antibiotic is placed on a nutrient agar, such as Mueller-Hinton agar, that has been covered with a broth containing a known concentration of the test bacterium. The test bacterium is a recent growth of a single bacterial clone. The antibiotic in the disk will diffuse into the agar along a decreasing concentration gradient. After a period of incubation for bacterial growth that is 18 to 24 hours for rapidly growing bacteria. The diameter of the zone of inhibition (ZOI) of bacterial growth is measured using a ruler or calliper. This zone is interpreted using clinical breakpoint values that have been predetermined using knowledge of the ZOI of susceptible bacteria, the antibiotic concentration curves in different tissues of target animal species as well as the maximum concentration a drug can safely reach at the site of infection. The isolate is then interpreted as resistant, intermediate or susceptible. This data is published and updated annually by organisations such as the Clinical and Laboratory Standards Institute, USA (CLSI) and European Committee on Antimicrobial Susceptibility Testing (EUCAST).
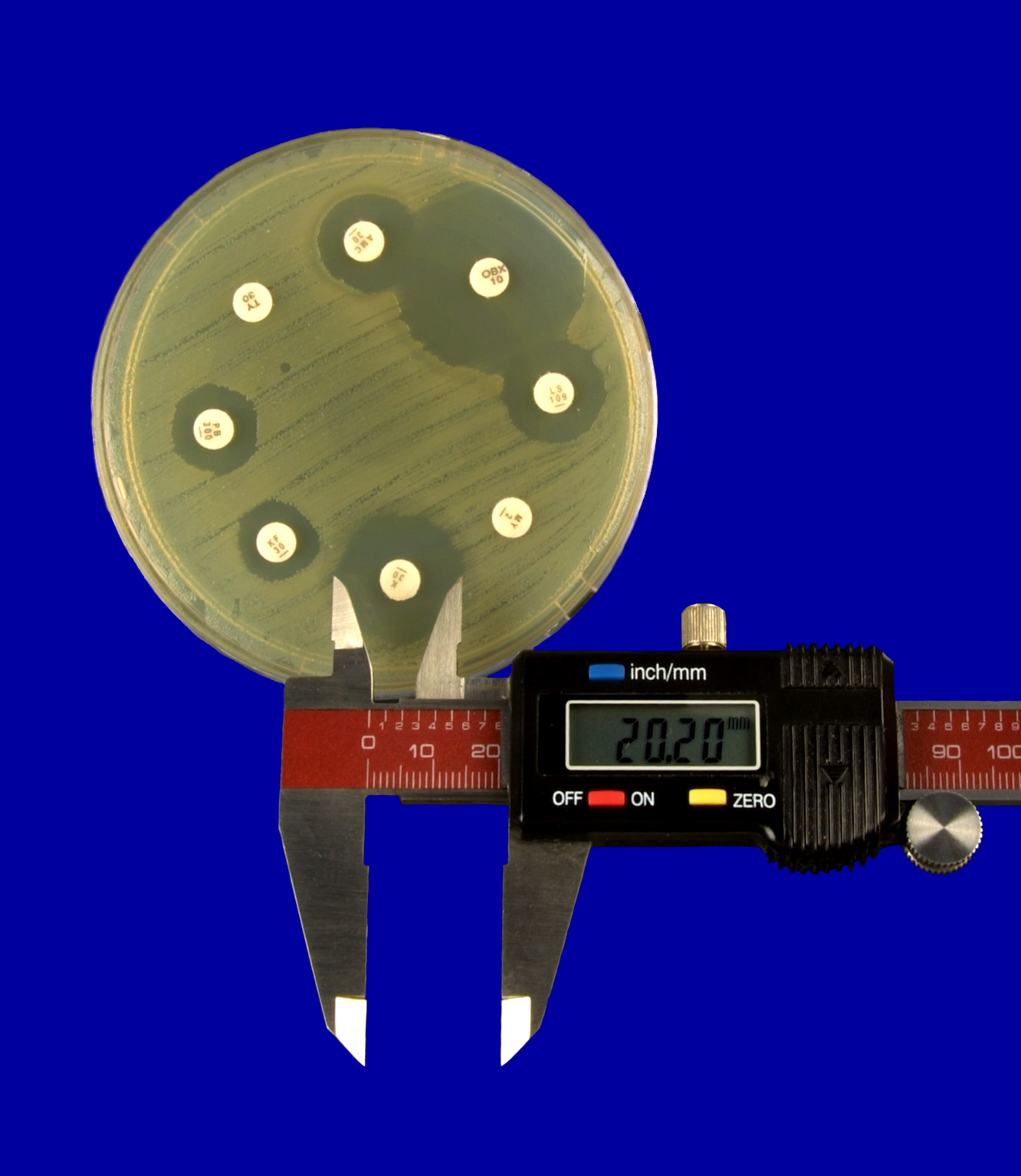
Test advantages:
- Allows a customised range of antibiotics to be tested
- Can see when there is a mixed bacterial population
- Is cheaper than the MIC
Test disadvantages:
- Is subject to user variation i.e. bacterial concentration variation, agar thickness, antibiotic disk potency loss. Thus it is important that these tests always be done with a known bacterium that has a known ZOI for each of the antibiotics tested. This is the so-called quality control bacterium. The test is considered successful if the ZOI of the QC bacterium is within its expected range.
- Is not reproducible or validated for many fastidious and slow-growing microbes.
Tips on how to measure the ZOI. Avoid measuring the ZOI between disks as different antibiotics may interact (be synergistic or antagonistic) where they meet in the agar.
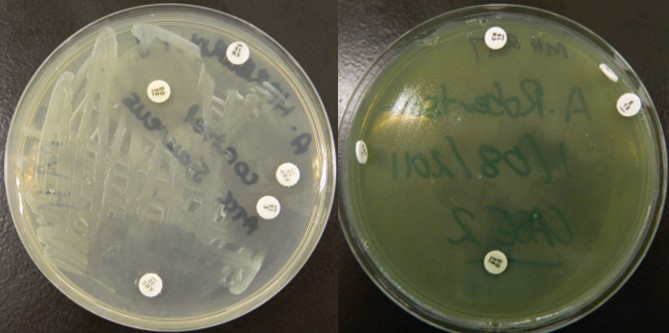
Minimum Inhibitory Concentration (MIC) Test
In this test a known concentration of bacteria are incubated with a dilution series of antibiotics. This is usually carried out in microtitre plates. Where bacterial growth is indicated by cloudiness of the antibiotic/bacterial/broth suspension. In U-bottomed plates the bacterial growth will sediment to form a “button”. Alternatively, indicators of bacterial growth i.e. broth reduction or sugar fermentation can be added to the suspension. The lowest concentration of antibiotic that inhibits the bacterial growth is known as the minimum inhibitory concentration (MIC). Like the disk diffusion tests, CLSI and EUCAST provide clinical breakpoint values.
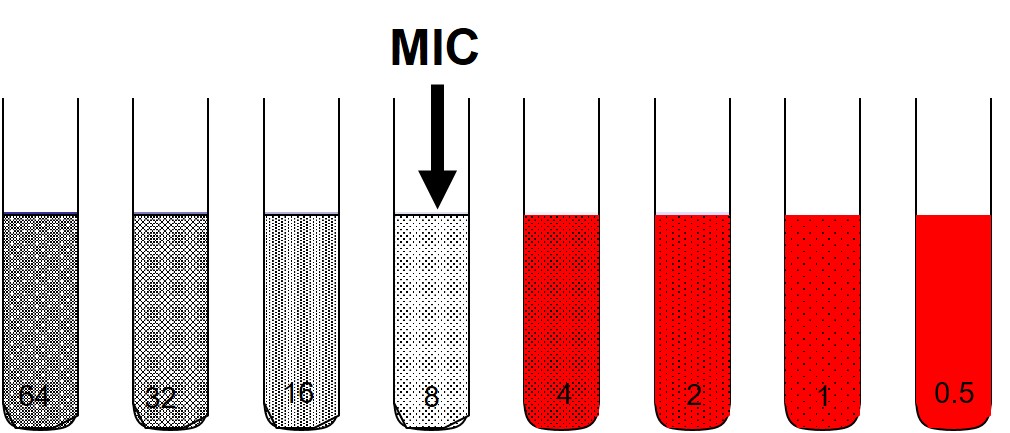

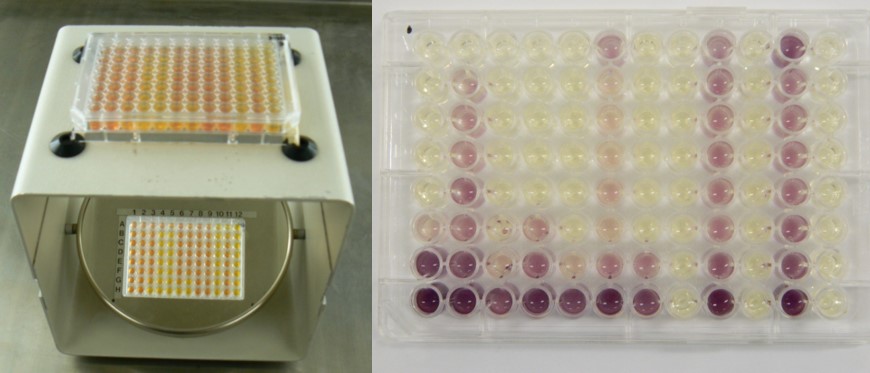
Advantages of the MIC
- For some bacteria i.e. mycoplasmas, anaerobes and fungi – where disk diffusion has not been validated or is not reproducible.
- When there is a high level of resistance and antibiotics may have to be used at doses that are higher than the manufacturer’s recommended dose or when animal patients have renal or liver disease where antibiotics are not metabolised or eliminated rapidly.
- To monitor resistance trends (surveillance programmes)
- Less inter-laboratory variation – more robust test
Disadvantages of the MIC
- Commercial MIC tests may not have all the antibiotics required and the antibiotic concentration range is limited, so may not include the clinical break point concentrations for all bacteria.
- More expensive than disk diffusion
- Can be harder to read
- May not note that there is not a single clone of bacteria and thus results may become unreliable
Agar gradient diffusion test (The E-test), an adapted disk diffusion test
The E-test using a concentration gradient strip containing the target antibiotic instead of a disk, combines the advantages of the disk diffusion and MIC tests. However, they are expensive and are only used when the clinician has to modify the dose of a particular antibiotic for an animal patient that has renal or liver disease. it has also been validated for fastidious anaerobes and mycoplasmas. The disk diffusion test is unreliable for the mentioned bacteria.
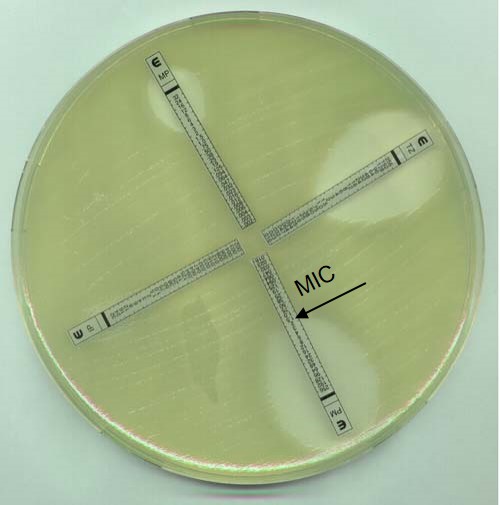
Tests for specific resistances
1. Testing for methicillin-resistant Staphylococcus aureus (MRSA), Staphylococcus pseudintermedius (MRSP) and coagulase negative Staphylococcus spp. (MRS)
Direct screening of samples: Only done when there are individuals at high risk of nosocomial infections.
- Culture on a selective agar.
- PCR to detection of the mecA and blaZ genes
Indirect detection: Culture of Staphylococcus spp. from the sample and then detection of methicillin resistance using one of the following:
- Edge test using a penicillin G disk. Sharp edge positive, fuzzy edge negative.
- Antibiotics: Oxacillin for MRSP, Cefoxitin for MRSA and MRS
- Rapid latex agglutination test to detect PBP2a antigen – only for mecA resistance
- PCR to detect mecA and blaZ genes
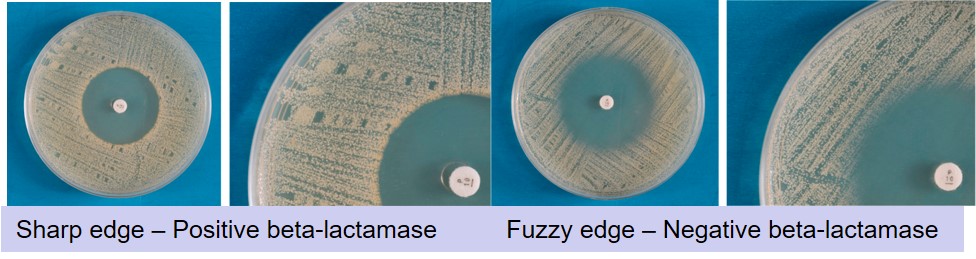
In an outbreak – tracing the spread or source of MRS.
Genetic fingerprinting methods can trace the source of the resistant bacteria. Methods used for Staphylococcus include the following:
- Pulsed-field gel electrophoresis (PFGEP) using the restriction enzyme Sma1. It discriminates the most.
- SCCmec typing (mPCR)
- Multilocus sequence typing (MLST) using 7 housekeeping genes
- Spa typing – single locus sequencing – the simplest method
2. Extended-spectrum beta-lactamase production in the gram-negative bacteria
To screen for the presence of ESBLsExtended-spectrum beta-lactamases (ES: Resistance to third-generation cephalosporins: ceftazidime or cefapodoxime and susceptibility to these antibiotics with ceftazidime or cefapodoxime with clavulanic acid.
For epidemiological purposes the resistance genes involved can be detected using qPCR i.e. The first ESBL detected was TEM-1 and TEM-2 which conferred resistance of gram-negative bacteria to penicillins and narrow-spectrum (first generation) cephalosporins.
Controlling antimicrobial resistance
Learning Objectives
Design a “Prudent Use of Antibiotics” program that is associated with knowledge of what drives antibiotic resistance and how it is disseminated.
What can veterinary practitioners do to reduce the emergence of antimicrobial resistance? = Prudent use of antibiotics
A. Work with clients to avoid the need for antimicrobials
- Regular health checks and screen animals for highly transmissible infectious agents
- Integrated disease control programmes, including vaccination, biosecurity, sanitation, testing for disease/highly transmissible agents, closed herds, quarantineof new animals, isolating susceptible animals, disease-free herds.
- Isolate infected animals or quarantine infected herds
- Good infection control programme in veterinary clinics and hospitals
B. Avoid inappropriate use of antimicrobials
- Develop protocols in your practice for antimicrobial use, including the tier system. These should be regularly monitored.
- Where possible select topical over systemic antibiotic therapy i.e. otitis externa should only be treated with topical antibiotics.
- When treating empirically, give preference to 1st tier antibiotics (See Table below) – those antibiotics where the consequences of antibiotic resistance development is less serious and there are alternative antibiotics. Where possible avoid the use of classes of antibiotic that are used to treat resistant bacterial in humans i.e. vancomycin which is used to treat MRSA and resistant Enterococcus species. Ciprofloxacin is also listed as high importance in humans, a similar drug enrofloxacin is registered for companion animal use. Ceftiofur, a 3rd generation cephalosporin is also listed as a highly important drug. So these antibiotics are usually 2nd or 3rd tier antibiotics.
- Educate animal owners to store and administer treatments properly and find alternative dosing protocols if owner compliance may be an issue i.e. the use of an injectable long-acting drug rather than oral dosing
- Avoid under-dosing. Use the most suitable antibiotic at the correct dose so that all the bacteria are destroyed (minimum of 7-10 days and 4 – 5 days post resolution of fever). Administer fluoroquinolones at doses greater than the MPCMutation Prevention Concentration (MPC) until 72 hours after the clinical signs have resolved
- Keep the course of antibiotics as short as possible, but ensure that target bacteria are destroyed.
C. Choose the right antimicrobial for the right microbe
- Identify the cause of disease and use specific treatments i.e. cephalexin a 1st generation cephalosporin, is not effective against Enterococcus.
- Use as narrow-spectrum of antibiotic as possible.
- Know the pharmacodynamicspharmacodynamics and pharmacokineticspharmacokinetics.
D. Monitor antimicrobial susceptibility
- Test for antimicrobial resistance especially if you are considering using 2nd and 3rd tier antibiotics.
- Monitor animal’s response to therapy
- Use results from antimicrobial surveillance programmes or laboratory reports on antimicrobial resistance trends. Note that the vast majority of cases submitted for antimicrobial susceptibility testing are likely to be treatment failures. Thus these reports may over-represent antimicrobial resistance.
E. Minimise Antimicrobial Use
- Only treat serious, confirmed bacterial or fungal infection where antibiotic therapy has a proven effect.
- Health status of the animal patient. For example. Acute diarrhoea in animals is usually self-limiting and therapy should be symptomatic rather than using antibiotics. However, immune-suppressed, those with systemic infection and those with serious co-morbities may still require antibiotic therapy.
- Use only registered antibiotics in food animals and ensure that the proper drug withholding period has been followed in the living animal before a product is sold for human consumption
- Replace antibiotics. Some cytokines or molecules promoting cytokine expression have been found to give as good a growth promoting effect as antibiotics in food animals.
F. Record and if necessary report
- Record any deviations from standard therapies
- Where appropriate, report suspected treatment failures
What should veterinarians do to prevent nosocomial infections with antibiotic resistant bacteria?
- Surveillance of high-risk animals
- Isolation of infected animals with minimum contact
- Restriction of antibiotic use
- Enhanced infection control
What should a country do to reduce the burden of antibiotic resistance?
- Active and Passive surveillance of common bacteria known to develop resistance in the medical, veterinary, agricultural and environmental sectors
- Antibiotic sales surveillance
- Publish antibiotic therapy guidelines for each animal species
- Regulation
- Education
- Research to improve the use of alternative or co-therapies. Alternative treatments such as bacteriophage therapy and the use of non-pathogenic bacteria to out compete resistant pathogenic bacteria (probiotics, faecal transplants) could also be considered. Combination therapy of antibiotics with AMPs, biofilm disruption enzymes e.g. amylases and DNAses, and quorum sensing inhibitors improve bacterial destruction in biofilms.
- Excellent biosecurity and preventative measures including vaccination.
A note on alternatives to antibiotics
- Bacteriophages. Virulent Phage cocktails containing a number of different phages have been proven to destroy their target bacteria and if availble they are a viable alternative to antibiotic therapy. However, their use is limited as they are highly bacteria specific requiring access to phage libraries. Bacteria can also quicly develop resistance to them.
- Probiotics: Probiotics are non-pathogenic bacteria that are administered orally to protect the intestinal tract and even other mucosae from harmful microorganisms. They have a number of beneficial effects. Refer to your notes on Bacterial pathogenesis – bacterial colonisation for more detail. Below is a diagram illustrating the primary benefically effects of these microorganisms. Since no single probiotic will provide all the beneficial effects, it is often recommended that a mixture of probiotics, that work synergistically, are administered to broaden their efficacy.
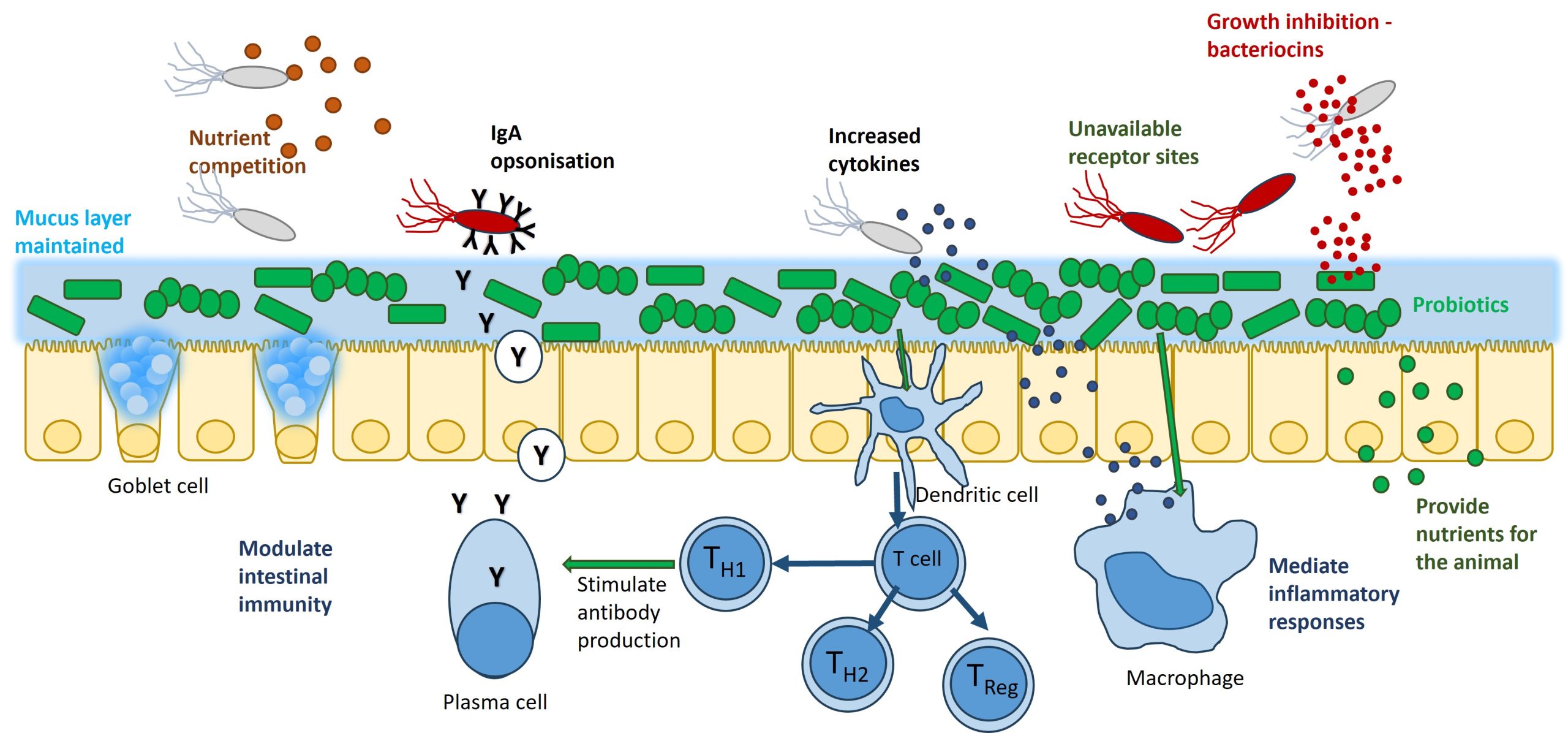
3.Prebiotics. Prebiotics are non-digestible sugars that undergo selective fermentation altering the composition and behavious of microorganisms. The are often administered with probiotics and are then knnown as synbiotics. Prebiotics nourish normal intestinal microflora that in turn provide nutrients for the animal and are better able to control pathogenic bacteria.
4. Antimicrobial peptides. Antimicrobial peptides are small molecules that are important in the innate immunity of the animal host. Many of these have proven to be inhibitory to pathogenic bacteria. However, before they can be used, more research has to be carried out on their toxicity to cells, better delivery systems, mechanisms of action and bioavailability.
5. Small molecules. These molecules have a narrow spectrum of activity and will either inhibit bacterial growth or virulence factor production. They can alsoo inhibit quorum sensing by bacteria which is needed for biofilm production. Many of these molecules are found naturally in plants i.e. grape seed extract, ginger or can be manufactured.
6. Short chain and medium chain organic acids. These are added to animal feed to reduce the colonisation of pathogenic Escherichia coli and Salmonella in the intestines of food animals. A limitation to their use is the fact that they are often digested before reaching the large intestine.
7. Essential oils like lemongrass, thyme and cinnamon oil can reduce bacterial growth in foods. However, care must be taken to avoid toxicity.
8. Host-directed therapies i.e. enhancing the host immune response. Some kinase inhibitors will improve the lysosomal activity of cells, increasing their ability to destroy intracellular bacteria.
This is an inherent characteristic of a particular species of microbe to a particular anti-infective agent.
When a particular microorganism obtains the ability to resist the activity of a particular antimicrobial to which it was previously susceptible.
The lowest concentration of antimicrobial required to stop the growth of the least susceptible bacterium in a large bacterial population.
A bacterium that has acquired resistance to at least one antibiotic in 3 or more antibiotic categories is considered to be multidrug resistant
The mechanisms microorganisms use to resist the action of antimicrobials.
A beta-lactam class of antibiotics that inhibit bacterial cell-wall synthesis
These are both transposons and and insertion nucleotide sequences that often carry gene cassettes of antibiotic resistance genes that can be transferred to bacterial plasmids and chromosomes.
A point mutation occurs in a genome when a single base pair is added, deleted or changed.
DNA topoisomerases are enzymes that catalyze changes in the topological state of DNA, interconverting relaxed and supercoiled forms, linked and unlinked species, and knotted and unknotted
Is an ansamycin that inhibits bacterial DNA-dependent RNA synthesis by inhibiting bacterial DNA-dependent RNA polymerase
It acts as a bacterial protein synthesis inhibitor
It is bactericidal and inhibits bacterial cell wall biogenesis by inactivating the enzyme MurA responsible for linking the building blocks of peptidoglycan
Resistance to other drugs within an antibiotic class or to unrelated drugs
Atopy is the genetic tendency to develop allergic diseases such as allergic rhinitis, asthma and atopic dermatitis (eczema).
Destruction or disruption (imbalance) of the normal microflora on or in the body. Example: It occurs when there is broad-spectrum antibiotic therapy
A long-acting 3rd generation cephalosporin with predominantly a gram-positive spectrum. Registered for skin and urinary tract infections in dogs and cats
Swine dysentery is a large intestine disease caused by the anaerobic spirochaete bacterium Brachyspira hyodysenteriae
Staphylococcus aureus and other staphylococci that have the mecA gene alter their penicillin binding proteins. This is observed as resistance to methicillin (or oxacillin, cefoxitin). These bacteria tend to be multidrug resistant.
Hospital-acquired infections. Clinical signs develop after 72 hours of hospitalisation.
Extended-spectrum beta-lactamases (ESBLs) are enzymes that confer resistance to most beta-lactam antibiotics, including penicillins, cephalosporins, and the monobactam aztreonam. Infections with ESBL-producing organisms have been associated with poor outcomes
Observable or expressed antimicrobial resistances
Procedures or measures designed to protect a population against harmful biological or biochemical substances.
A quarantine is a restriction on the movement of people, animals and goods which is intended to prevent the spread of infectious agents or pests
Effects of drugs and their mechanism of action
Movement of drugs within the body
Probiotics are live non-pathogenic microorganisms that are administered in large numbers to promote a healthy intestine.