7. Essential compounds of life

- Identify the building blocks of carbohydrates, lipids and proteins and describe how they join together
- Describe the structure of carbohydrates and identify the primary functions in the body
- Describe the structure of lipids and list the four types important in human functioning
- Describe the structure of proteins and list their functions in the body
- Describe how and where each of the nutrients are broken down to their basic units
- Explain how the basic units get from the gut into the bloodstream
The large molecules necessary for life are called biological macromolecules. There are four major classes of biological macromolecules (carbohydrates, lipids, proteins, and nucleic acids), and each is an important component of the cell, performing a wide array of functions. Combined, these molecules make up the majority of a cell’s mass. Macromolecules are organic, meaning that they contain carbon. In addition, they may contain hydrogen, oxygen, nitrogen, phosphorus, sulfur, and additional minor elements. Table 7.1 lists the four classes of biomolecules along with their major functions. Nucleic acids have been discussed in Chapter 6: Coding life so in this chapter, we will explore the structure of proteins, carbohydrate and lipids and discuss how these molecules are formed and the functions they fulfil.
Biomolecule | Building block | Function |
Protein | Amino acid | Fundamental component of structure and dynamic metabolic function in the cell |
Lipid | No single building block for all lipids | Long-term energy molecule, structural component of the cell membrane (phospholipids) |
Carbohydrate | Monosaccharides | Short and medium-term energy molecule, structure component for cell recognition |
Nucleic acids | Nucleotides | Hereditary information, protein synthesis |
7.1 | What makes a compound organic?
All biomolecules contain the elements carbon, hydrogen and oxygen. Nitrogen, sulfur and phosphorus can also be present. Elements have been discussed in detail in Chapter 3: Chemistry for life but we will briefly revise the properties of carbon before discussing the classes of biomolecules.
Carbon
It is often said that life is “carbon-based.” This means that carbon atoms, bonded to other carbon atoms or other elements, form the fundamental components of many, if not most, of the molecules found uniquely in living things. Other elements play important roles in biological molecules, but carbon certainly qualifies as the “foundation” element for molecules in living things. It is the bonding properties of carbon atoms that are responsible for its important role.
Carbon bonding
Carbon contains four electrons in its outer shell. Therefore, it can form four covalent bonds with other atoms or molecules. The simplest organic carbon molecule is methane (CH4), in which four hydrogen atoms bind to a carbon atom (Figure 7.2).

However, structures that are more complex are made using carbon. Any of the hydrogen atoms can be replaced with another carbon atom covalently bonded to the first carbon atom. In this way, long and branching chains of carbon compounds can be made (Figure 7.3a). The carbon atoms may bond with atoms of other elements, such as nitrogen, oxygen, and phosphorus (Figure 7.3b). The molecules may also form rings, which themselves can link with other rings (Figure 7.3c). This diversity of molecular forms accounts for the diversity of functions of the biological macromolecules and is based to a large degree on the ability of carbon to form multiple bonds with itself and other atoms.

What are the structure and function of carbohydrates?
Carbohydrates are macromolecules with which most consumers are somewhat familiar. To lose weight, some individuals adhere to low-carb diets. Athletes, in contrast, often carb-load before important competitions to ensure that they have sufficient energy to compete at a high level. Carbohydrates are, in fact, an essential part of our diet; grains, fruits, and vegetables are all natural sources of carbohydrates. Carbohydrates provide energy to the body, particularly through glucose, a simple sugar. Carbohydrates also have other important functions in humans, animals, and plants.
Carbohydrates can be represented by the formula (CH2O)n, where n is the number of carbon atoms in the molecule. In other words, the ratio of carbon to hydrogen to oxygen is 1:2:1 in carbohydrate molecules. Carbohydrates are classified into three subtypes: monosaccharides, disaccharides, and polysaccharides.
Monosaccharides (mono- = one; sacchar- = sweet) are simple sugars, the most common of which is glucose. In monosaccharides, the number of carbon atoms usually ranges from three to six. Most monosaccharide names end with the suffix -ose. Depending on the number of carbon atoms in the sugar, they may be known as trioses (three carbon atoms), pentoses (five carbon atoms), and hexoses (six carbon atoms).
Monosaccharides may exist as a linear chain or as ring-shaped molecules; in aqueous solutions, they are usually found in the ring form.
The chemical formula for glucose is C6H12O6. In most living species, glucose is an important source of energy. During cellular respiration, energy is released from glucose, and that energy is used to help make adenosine triphosphate (ATP). Plants synthesise glucose using carbon dioxide and water by the process of photosynthesis, and the glucose, in turn, is used for the energy requirements of the plant. The excess synthesised glucose is often stored as starch that is broken down by other organisms that feed on plants.
Galactose (part of lactose, or milk sugar) and fructose (found in fruit) are other common monosaccharides. Although glucose, galactose, and fructose all have the same chemical formula (C6H12O6), they differ structurally and chemically (and are known as isomers) because of differing arrangements of atoms in the carbon chain (Figure 7.4).

Disaccharides (di- = two) form when two monosaccharides undergo a dehydration reaction (a reaction in which the removal of a water molecule occurs). During this process, the hydroxyl group (–OH) of one monosaccharide combines with a hydrogen atom of another monosaccharide, releasing a molecule of water (H2O) and forming a covalent bond between atoms in the two sugar molecules.
Common disaccharides include lactose, maltose, and sucrose. Lactose is a disaccharide consisting of the monomers glucose and galactose. It is found naturally in milk. Maltose, or malt sugar, is a disaccharide formed from a dehydration reaction between two glucose molecules. The most common disaccharide is sucrose, or table sugar, which is composed of the monomers glucose and fructose.
A long chain of monosaccharides linked by covalent bonds is known as a polysaccharide (poly- = many). The chain may be branched or unbranched, and it may contain different types of monosaccharides. Polysaccharides may be very large molecules. Starch, glycogen, cellulose, and chitin are examples of polysaccharides.
Starch is the stored form of sugars in plants and is made up of amylose and amylopectin (both polymers of glucose). Plants are able to synthesise glucose, and the excess glucose is stored as starch in different plant parts, including roots and seeds. The starch that is consumed by animals is broken down into smaller molecules, such as glucose. The cells can then absorb the glucose.
Glycogen is the storage form of glucose in humans and other vertebrates and is made up of monomers of glucose. Glycogen is the animal equivalent of starch and is a highly branched molecule usually stored in liver and muscle cells. Whenever glucose levels decrease, glycogen is broken down to release glucose.
Cellulose is one of the most abundant natural biopolymers. The cell walls of plants are mostly made of cellulose, which provides structural support to the cell. Wood and paper are mostly cellulosic in nature. Cellulose is made up of glucose monomers that are linked by bonds between particular carbon atoms in the glucose molecule.
Every other glucose monomer in cellulose is flipped over and packed tightly as extended long chains. This gives cellulose its rigidity and high tensile strength—which is so important to plant cells. Cellulose passing through our digestive system is called dietary fibre. While the glucose-glucose bonds in cellulose cannot be broken down by human digestive enzymes, herbivores such as cows, buffalos, and horses are able to digest grass that is rich in cellulose and use it as a food source. In these animals, certain species of bacteria reside in the rumen (part of the digestive system of herbivores) and secrete the enzyme cellulase. The appendix also contains bacteria that break down cellulose, giving it an important role in the digestive systems of ruminants. Cellulases can break down cellulose into glucose monomers that can be used as an energy source by the animal.
Carbohydrates serve other functions in different animals. Arthropods, such as insects, spiders, and crabs, have an outer skeleton, called the exoskeleton, which protects their internal body parts. This exoskeleton is made of the biological macromolecule chitin, which is a nitrogenous carbohydrate. It is made of repeating units of a modified sugar containing nitrogen.
Thus, through differences in molecular structure, carbohydrates are able to serve the very different functions of energy storage (starch and glycogen) and structural support and protection (cellulose and chitin) (Figure 7.5).

Career Connection: Registered Dietitian
Obesity is a worldwide health concern, and many diseases, such as diabetes and heart disease, are becoming more prevalent because of obesity. This is one of the reasons why registered dietitians are increasingly sought after for advice. Registered dietitians help plan food and nutrition programs for individuals in various settings. They often work with patients in healthcare facilities, designing nutrition plans to prevent and treat diseases. For example, dietitians may teach a patient with diabetes how to manage blood-sugar levels by eating the correct types and amounts of carbohydrates. Dietitians may also work in nursing homes, schools, and private practices.
To become a registered dietitian, one needs to earn at least a bachelor’s degree in dietetics, nutrition, food technology, or a related field. In addition, registered dietitians must complete a supervised internship program and pass a national exam. Those who pursue careers in dietetics take courses in nutrition, chemistry, biochemistry, biology, microbiology, and human physiology. Dietitians must become experts in the chemistry and functions of food (proteins, carbohydrates, and fats).
What are the structure and functions of lipids?
Lipids include a diverse group of compounds that are united by a common feature. Lipids are hydrophobic (water-fearing), or insoluble in water, because they are nonpolar molecules. This is because they are hydrocarbons that include only nonpolar carbon-carbon or carbon-hydrogen bonds. Lipids perform many different functions in a cell. Cells store energy for long-term use in the form of lipids called fats. Lipids also provide insulation from the environment for plants and animals (Figure 7.6). For example, they help keep aquatic birds and mammals dry because of their water-repelling nature. Lipids are also the building blocks of many hormones and are an important constituent of the plasma membrane. Lipids include fats, oils, waxes, phospholipids, and steroids.

Triglycerides
A fat molecule, such as a triglyceride, consists of two main components—glycerol and fatty acids. Glycerol is an organic compound with three carbon atoms, five hydrogen atoms, and three hydroxyl (–OH) groups. Fatty acids have a long chain of hydrocarbons to which an acidic carboxyl group is attached, hence the name fatty acid. The number of carbons in the fatty acid may range from 4 to 36; most common are those containing 12–18 carbons. In a fat molecule, a fatty acid is attached to each of the three oxygen atoms in the –OH groups of the glycerol molecule with a covalent bond (Figure 7.7).

During this covalent bond formation, three water molecules are released. The three fatty acids in the fat may be similar or dissimilar. These fats are also called triglycerides because they have three fatty acids. Some fatty acids have common names that specify their origin. For example, palmitic acid, a saturated fatty acid, is derived from the palm tree.
Fatty acids may be saturated or unsaturated. In a fatty acid chain, if there are only single bonds between neighbouring carbons in the hydrocarbon chain, the fatty acid is saturated. Saturated fatty acids are saturated with hydrogen; in other words, the number of hydrogen atoms attached to the carbon skeleton is maximised.
When the hydrocarbon chain contains a double bond, the fatty acid is an unsaturated fatty acid.
Most unsaturated fats are liquid at room temperature and are called oils. If there is one double bond in the molecule, then it is known as a monounsaturated fat (e.g., olive oil), and if there is more than one double bond, then it is known as a polyunsaturated fat (e.g., canola oil).
Saturated fats tend to get packed tightly and are solid at room temperature. Animal fats with stearic acid and palmitic acid contained in meat, and the fat with butyric acid contained in butter, are examples of saturated fats. Mammals store fats in specialised cells called adipocytes, where globules of fat occupy most of the cell. In plants, fat or oil is stored in seeds and is used as a source of energy during embryonic development.
Unsaturated fats or oils are usually of plant origin and contain unsaturated fatty acids. The double bond causes a bend or a kink that prevents the fatty acids from packing tightly, keeping them liquid at room temperature. Olive oil, corn oil, canola oil, and cod liver oil are examples of unsaturated fats. Unsaturated fats help to improve blood cholesterol levels, whereas saturated fats contribute to plaque formation in the arteries, which increases the risk of a heart attack.
In the food industry, oils are artificially hydrogenated to make them semi-solid, leading to less spoilage and increased shelf life. Simply speaking, hydrogen gas is bubbled through oils to solidify them. During this hydrogenation process, double bonds of the cis-conformation in the hydrocarbon chain may be converted to double bonds in the trans-conformation. This forms a trans-fat from a cis-fat. The orientation of the double bonds affects the chemical properties of the fat (Figure 7.8).

Margarine, some types of peanut butter, and shortening are examples of artificially hydrogenated trans-fats. Recent studies have shown that an increase in trans-fats in the human diet may lead to an increase in levels of low-density lipoprotein (LDL), or “bad” cholesterol, which, in turn, may lead to plaque deposition in the arteries, resulting in heart disease. Many fast-food restaurants have recently eliminated the use of trans-fats, and U.S. food labels are now required to list their trans-fat content (this is not the case in Australia see Dietitians Australia for more information).
Essential fatty acids are fatty acids that are required but not synthesised by the human body. Consequently, they must be supplemented through the diet. Omega-3 fatty acids fall into this category and are one of only two known essential fatty acids for humans (the other being omega-6 fatty acids). They are a type of polyunsaturated fat and are called omega-3 fatty acids because the third carbon from the end of the fatty acid participates in a double bond.
Salmon, trout, and tuna are good sources of omega-3 fatty acids. Omega-3 fatty acids are important in brain function and normal growth and development. They may also prevent heart disease and reduce the risk of cancer.
Like carbohydrates, fats have received a lot of bad publicity. It is true that eating an excess of fried foods and other fatty foods leads to weight gain. However, fats do have important functions. Fats serve as long-term energy storage. They also provide insulation for the body. Therefore, healthy unsaturated fats in moderate amounts should be consumed on a regular basis.
Phospholipids
Phospholipids are the major constituent of the plasma membrane. Like fats, they are composed of fatty acid chains attached to a glycerol or similar backbone. Instead of three fatty acids attached, however, there are two fatty acids, and the third carbon of the glycerol backbone is bound to a phosphate group. The phosphate group is modified by the addition of an alcohol.
A phospholipid has both hydrophobic and hydrophilic regions. The fatty acid chains are hydrophobic and exclude themselves from water, whereas the phosphate is hydrophilic and interacts with water.
Cells are surrounded by a membrane, which has a bilayer of phospholipids. The fatty acids of phospholipids face inside, away from water, whereas the phosphate group can face either the outside environment or the inside of the cell, which are both aqueous.
Steroids and waxes
Unlike the phospholipids and fats discussed earlier, steroids have a ring structure. Although they do not resemble other lipids, they are grouped with them because they are also hydrophobic. All steroids have four, linked carbon rings and several of them, like cholesterol, have a short tail.
Cholesterol is a steroid. Cholesterol is mainly synthesised in the liver and is the precursor of many steroid hormones, such as testosterone and oestradiol. It is also the precursor of vitamins E and K. Cholesterol is the precursor of bile salts, which help in the breakdown of fats and their subsequent absorption by cells. Although cholesterol is often spoken of in negative terms, it is necessary for the proper functioning of the body. It is a key component of the plasma membranes of animal cells.
Waxes are made up of a hydrocarbon chain with an alcohol (–OH) group and a fatty acid. Examples of animal waxes include beeswax and lanolin. Plants also have waxes, such as the coating on their leaves, that helps prevent them from drying out.
What are the structure and function of proteins?
Proteins are one of the most abundant organic molecules in living systems and have the most diverse range of functions of all macromolecules. Proteins may be structural, regulatory, contractile, or protective; they may serve in transport, storage, or membranes; or they may be toxins or enzymes. Each cell in a living system may contain thousands of different proteins, each with a unique function. Their structures, like their functions, vary greatly. They are all, however, polymers of amino acids, arranged in a linear sequence.
The functions of proteins are very diverse because there are 20 different chemically distinct amino acids that form long chains, and the amino acids can be in any order. For example, proteins can function as enzymes or hormones. Enzymes, which are produced by living cells, are catalysts in biochemical reactions (like digestion) and are usually proteins. Each enzyme is specific for the substrate (a reactant that binds to an enzyme) upon which it acts. Enzymes can function to break molecular bonds, to rearrange bonds, or to form new bonds. An example of an enzyme is salivary amylase, which breaks down amylose, a component of starch.
Hormones are chemical signaling molecules, usually proteins or steroids, secreted by an endocrine gland or group of endocrine cells that act to control or regulate specific physiological processes, including growth, development, metabolism, and reproduction. For example, insulin is a protein hormone that maintains blood glucose levels.
Proteins have different shapes and molecular weights; some proteins are globular in shape whereas others are fibrous in nature. For example, haemoglobin is a globular protein, but collagen, found in our skin, is a fibrous protein. Protein shape is critical to its function. Changes in temperature, pH, and exposure to chemicals may lead to permanent changes in the shape of the protein, leading to a loss of function or denaturation (to be discussed in more detail later). All proteins are made up of different arrangements of the same 20 kinds of amino acids.
Amino acids are the monomers that make up proteins. Each amino acid has the same fundamental structure, which consists of a central carbon atom bonded to an amino group (–NH2), a carboxyl group (–COOH), and a hydrogen atom. Every amino acid also has another variable atom or group of atoms bonded to the central carbon atom known as the R group. The R group is the only difference in structure between the 20 amino acids; otherwise, the amino acids are identical (Figure 7.9).

The chemical nature of the R group determines the chemical nature of the amino acid within its protein (that is, whether it is acidic, basic, polar, or nonpolar).
The sequence and number of amino acids ultimately determine a protein’s shape, size, and function. Each amino acid is attached to another amino acid by a covalent bond, known as a peptide bond, which is formed by a dehydration reaction. The carboxyl group of one amino acid and the amino group of a second amino acid combine, releasing a water molecule. The resulting bond is the peptide bond.
The products formed by such a linkage are called polypeptides. While the terms polypeptide and protein are sometimes used interchangeably, a polypeptide is technically a polymer of amino acids, whereas the term protein is used for a polypeptide or polypeptides that have combined together, have a distinct shape, and have a unique function.
Protein structure
As mentioned earlier, the shape of a protein is critical to its function. Recall from Chapter 6: Coding life, the order of the chain of amino acids is determined by the gene and is the primary structure of the protein. The relative position of the amino acids to each other allows hydrogen bonding and other types of internal bonding to occur, which gives the protein its secondary and tertiary structures. Finally, the quaternary structure is formed when a functional protein is made up of more than one tertiary protein (Figure 7.10).

Crossword puzzle
7.2 | How do we get nutrients into the cells?
All living organisms need nutrients to survive. While plants can obtain nutrients from their roots and the energy molecules required for cellular function through the process of photosynthesis, animals obtain their nutrients by the consumption of other organisms. At the cellular level, the biological molecules necessary for animal function are amino acids, lipid molecules, nucleotides, and simple sugars. However, the food consumed consists of protein, fat, and complex carbohydrates. Animals must convert these macromolecules into the simple molecules required for maintaining cellular function. The conversion of the food consumed to the nutrients required is a multistep process involving digestion and absorption. During digestion, food particles are broken down to smaller components, which are later absorbed by the body. This happens by both physical/mechanical means, such as chewing, and by chemical means. One of the challenges in human nutrition is maintaining a balance between food intake, storage, and energy expenditure. Taking in more food energy than is used in activity leads to storage of the excess in the form of fat deposits. The rise in obesity and the resulting diseases like type 2 diabetes makes understanding the role of diet and nutrition in maintaining good health all the more important.
Watch this TED-Ed video [4:57] for an overview of the digestion of food in different regions of the digestive tract. Note the route of non-fat nutrients from the small intestine to their release as nutrients to the body.
How is the digestive system organised?
The process of digestion begins in the mouth with the intake of food. The teeth play an important role in masticating (chewing) or physically breaking food into smaller particles. The enzymes present in saliva also begin to chemically break down food. The food is then swallowed and enters the oesophagus—a long tube that connects the mouth to the stomach. Using peristalsis, or wave-like smooth-muscle contractions, the muscles of the oesophagus push the food toward the stomach. The stomach contents are extremely acidic, with a pH between 1.5 and 2.5. This acidity kills microorganisms, breaks down food tissues, and activates digestive enzymes. Further breakdown of food takes place in the small intestine where bile produced by the liver, and enzymes produced by the small intestine and the pancreas, continue the process of digestion. The smaller molecules are absorbed into the bloodstream through the epithelial cells lining the walls of the small intestine. The waste material travels on to the large intestine where water is absorbed and the drier waste material is compacted into faeces; it is stored until it is excreted through the anus.

Oral cavity
Both mechanical and chemical digestion begin in the mouth or oral cavity, which is the point of entry of food into the digestive system. The food is broken into smaller particles by mastication, the chewing action of the teeth. All mammals have teeth and can chew their food to begin the process of physically breaking it down into smaller particles.
The chemical process of digestion begins during chewing as food mixes with saliva, produced by the salivary glands (Figure 7.12). Saliva contains mucus that moistens food and buffers the pH of the food. Saliva also contains lysozyme, which has antibacterial action. It also contains an enzyme called salivary amylase that begins the process of converting starches in the food into a disaccharide called maltose. Another enzyme called lipase is produced by cells in the tongue to break down fats. The chewing and wetting action provided by the teeth and saliva prepare the food into a mass called the bolus for swallowing. The tongue helps in swallowing—moving the bolus from the mouth into the pharynx. The pharynx opens to two passageways: the oesophagus and the trachea. The oesophagus leads to the stomach and the trachea leads to the lungs. The epiglottis is a flap of tissue that covers the tracheal opening during swallowing to prevent food from entering the lungs.
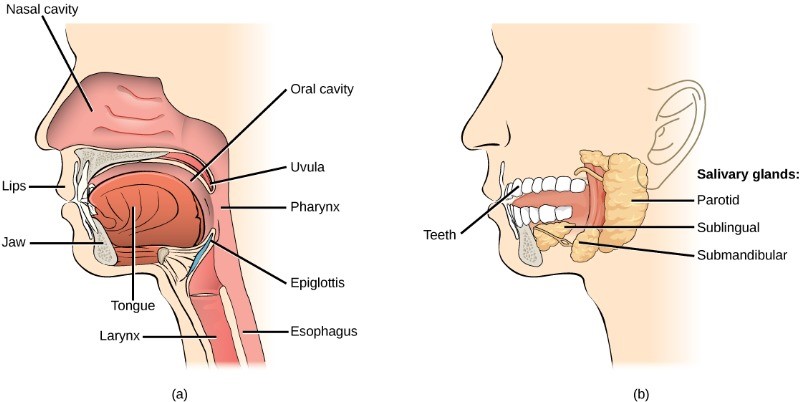
Oesophagus
The oesophagus is a tubular organ that connects the mouth to the stomach. The chewed and softened food passes through the oesophagus after being swallowed. The smooth muscles of the oesophagus undergo peristalsis that pushes the food toward the stomach. The peristaltic wave is unidirectional—it moves food from the mouth the stomach, and reverse movement is not possible, except in the case of the vomit reflex. The peristaltic movement of the oesophagus is an involuntary reflex; it takes place in response to the act of swallowing.
Ring-like muscles called sphincters form valves in the digestive system. The gastro-oesophageal sphincter (or cardiac sphincter) is located at the stomach end of the oesophagus. In response to swallowing and the pressure exerted by the bolus of food, this sphincter opens, and the bolus enters the stomach. When there is no swallowing action, this sphincter is shut and prevents the contents of the stomach from travelling up the oesophagus. Acid reflux or “heartburn” occurs when the acidic digestive juices escape into the oesophagus.
Stomach
A large part of protein digestion occurs in the stomach. The stomach is a saclike organ that secretes gastric digestive juices.
Protein digestion is carried out by an enzyme called pepsin in the stomach chamber. The highly acidic environment kills many microorganisms in the food and, combined with the action of the enzyme pepsin, results in the catabolism of protein in the food. Chemical digestion is facilitated by the churning action of the stomach caused by contraction and relaxation of smooth muscles. The partially digested food and gastric juice mixture is called chyme. Gastric emptying occurs within two to six hours after a meal. Only a small amount of chyme is released into the small intestine at a time. The movement of chyme from the stomach into the small intestine is regulated by hormones, stomach distension and muscular reflexes that influence the pyloric sphincter (the valve between the stomach and the small intestine).
The stomach lining is unaffected by pepsin and the acidity because pepsin is released in an inactive form and the stomach has a thick mucus lining that protects the underlying tissue.
Small intestine
Chyme moves from the stomach to the small intestine. The small intestine is the organ where the digestion of protein, fats, and carbohydrates is completed, and also where the majority of absorption occurs. The small intestine is a long tube-like organ with a highly folded surface containing finger-like projections called the villi (Figure 7.13). The top surface of each villus has many microscopic projections called microvilli. The epithelial cells of these structures absorb nutrients from the digested food and release them to the bloodstream on the other side. The villi and microvilli, with their many folds, increase the surface area of the small intestine and increase absorption efficiency of the nutrients.

The human small intestine is over 6 m (19.6ft) long and is divided into three parts: the duodenum, the jejunum and the ileum (Figure 7.14). The duodenum is separated from the stomach by the pyloric sphincter. The chyme is mixed with pancreatic juices, an alkaline solution rich in bicarbonate that neutralises the acidity of chyme from the stomach. Pancreatic juices contain several digestive enzymes that break down starches, disaccharides, proteins, and fats. Bile is produced in the liver and stored and concentrated in the gallbladder; it enters the duodenum through the bile duct. Bile contains bile salts, which make lipids accessible to the water-soluble enzymes. The monosaccharides, amino acids, bile salts, vitamins, and other nutrients are absorbed by the cells of the intestinal lining.

The undigested food is sent to the colon from the ileum via peristaltic movements. The ileum ends and the large intestine begins at the ileocecal valve. The vermiform, worm-like appendix is located at the ileocecal valve/sphincter (Figure 7.16). The appendix of humans has a minor role in immunity.
Large intestine
The large intestine reabsorbs the water from indigestible food material and processes the waste material (Figure 7.15). The human large intestine is much smaller in length compared to the small intestine but larger in diameter. It has three parts: the cecum, the colon, and the rectum. The cecum joins the ileum to the colon and is the receiving pouch for the waste matter. The colon is home to many bacteria or intestinal flora that aid in the digestive processes. The colon has four regions, the ascending colon, the transverse colon, the descending colon and the sigmoid colon. The main functions of the colon are to extract the water and mineral salts from undigested food, and to store waste material.

The rectum (Figure 7.15) stores faeces until defecation. The faeces are propelled using peristaltic movements during elimination. The anus is an opening at the far end of the digestive tract and is the exit point for the waste material. Two sphincters regulate the exit of faeces, the inner sphincter is involuntary and the outer sphincter is voluntary.
Accessory organs
The organs discussed above are the organs of the digestive tract through which food passes. Accessory organs add secretions and enzymes that break down food into nutrients. Accessory organs include the salivary glands, the liver, the pancreas, and the gall bladder. The secretions of the liver, pancreas, and gallbladder are regulated by hormones in response to food consumption. The liver is the largest internal organ in humans and it plays an important role in digestion of fats and detoxifying blood. The liver produces bile, a digestive juice that is required for the breakdown of fats in the duodenum. The liver also processes the absorbed vitamins and fatty acids and synthesises many plasma proteins. The gallbladder is a small organ that aids the liver by storing bile and concentrating bile salts. The pancreas lies transversely behind and below the stomach and is made up of both exocrine and endocrine tissue (Figure 7.16). The exocrine part secretes pancreatic juice, which includes bicarbonate that neutralises the acidic chyme, and a variety of enzymes for the digestion of protein and carbohydrates. The duct from the pancreas merges with the common bile duct and delivers the pancreatic juice to the duodenum.

The endocrine function of the pancreas will be discussed further in Chapter 10: Messages in the body.
What are the processes of digestion?
The processes of digestion include six activities: ingestion, propulsion, mechanical or physical digestion, chemical digestion, absorption, and defecation. The first of these processes, ingestion, refers to the entry of food into the alimentary canal through the mouth. As discussed previously, the food is chewed and mixed with saliva. Food leaves the mouth when the tongue and pharyngeal muscles propel it into the oesophagus. This act of swallowing, the last voluntary act until defecation, is an example of propulsion, which refers to the movement of food through the digestive tract. It includes both the voluntary process of swallowing and the involuntary process of peristalsis. These waves also play a role in mixing food with digestive juices. Peristalsis is so powerful that foods and liquids you swallow enter your stomach even if you are standing on your head.
Digestion includes both mechanical and chemical processes:
Mechanical digestion is a purely physical process that does not change the chemical nature of the food. Instead, it makes the food smaller to increase both surface area and mobility. It includes mastication, or chewing, as well as tongue movements that help break food into smaller bits and mix food with saliva. Although there may be a tendency to think that mechanical digestion is limited to the first steps of the digestive process, it occurs after the food leaves the mouth, as well. The mechanical churning of food in the stomach serves to further break it apart and expose more of its surface area to digestive juices, creating an acidic “soup” called chyme. Further mechanical digestion, known as segmentation, occurs in the small intestine.
In chemical digestion, starting in the mouth, digestive secretions break down complex food molecules into their chemical building blocks (for example, proteins into separate amino acids). These secretions vary in composition, but typically contain water, various enzymes, acids, and salts. The process begins in the mouth, continues in the stomach and is completed in the small intestine. Food that has been broken down is of no value to the body unless it enters the bloodstream and its nutrients are put to work. This occurs through the process of absorption, which takes place primarily within the small intestine. There, most nutrients are absorbed from the lumen of the alimentary canal into the bloodstream through the epithelial cells that make up the mucosa. Lipids are absorbed into lacteals and are transported via the lymphatic vessels to the bloodstream (the subclavian veins near the heart). In defecation, the final step in digestion, undigested materials are removed from the body as faeces.
In some cases, a single organ is in charge of a digestive process. For example, ingestion occurs only in the mouth and defecation only in the anus. However, most digestive processes involve the interaction of several organs and occur gradually as food moves through the alimentary canal (Figure 7.17).

How are each of the macromolecules digested and absorbed?
As you have learned, the process of mechanical digestion is relatively simple. It involves the physical breakdown of food but does not alter its chemical makeup. Chemical digestion, on the other hand, is a complex process that reduces food into its chemical building blocks, which are then absorbed to nourish the cells of the body (Figure 7.18). In this section, you will look more closely at the processes of chemical digestion and absorption.

Chemical digestion
Large food molecules (for example, proteins, lipids, nucleic acids, and starches) must be broken down into subunits that are small enough to be absorbed by the lining of the alimentary canal. This is accomplished by enzymes through hydrolysis.
Carbohydrate digestion
The average American diet is about 50 percent carbohydrates, which may be classified according to the number of monomers they contain of simple sugars (monosaccharides and disaccharides) and/or complex sugars (polysaccharides). Glucose, galactose, and fructose are the three monosaccharides that are commonly consumed and are readily absorbed. Your digestive system is also able to break down the disaccharide sucrose (regular table sugar: glucose + fructose), lactose (milk sugar: glucose + galactose), and maltose (grain sugar: glucose + glucose), and the polysaccharides glycogen and starch (chains of monosaccharides). Your bodies do not produce enzymes that can break down most fibrous polysaccharides, such as cellulose. While indigestible polysaccharides do not provide any nutritional value, they do provide dietary fibre, which helps propel food through the alimentary canal.
The chemical digestion of starches begins in the mouth with the salivary enzyme amylase and has been reviewed above.
In the small intestine, pancreatic amylase continues the breakdown starch and carbohydrate digestion that was started in the mouth (Figure 7.19). After amylases break down starch into smaller fragments, three small intestine enzymes hydrolyse sucrose, lactose, and maltose into monosaccharides. Sucrase splits sucrose into one molecule of fructose and one molecule of glucose; maltase breaks down maltose and maltotriose into two and three glucose molecules, respectively; and lactase breaks down lactose into one molecule of glucose and one molecule of galactose. Insufficient lactase can lead to lactose intolerance.

Protein digestion
Proteins are polymers composed of amino acids linked by peptide bonds to form long chains. Digestion reduces them to their constituent amino acids. You usually consume about 15 to 20 percent of your total calorie intake as protein.
The enzymes that break down proteins are known collectively as proteases and peptidases, they sometimes end in the suffix -in rather than -ase. The digestion of protein starts in the stomach, where HCl and pepsin break proteins into smaller polypeptides, which then travel to the small intestine (Figure 7.20). Chemical digestion in the small intestine is continued by pancreatic enzymes, including chymotrypsin and trypsin, each of which act on specific bonds in amino acid sequences. At the same time, the cells of the small intestine secrete enzymes such as aminopeptidase and dipeptidase, which further break down peptide chains. This results in molecules small enough to enter the bloodstream (Figure 7.21).


Lipid digestion
A healthy diet limits lipid intake to 35 percent of total calorie intake. The most common dietary lipids are triglycerides, which are made up of a glycerol molecule bound to three fatty acid chains. Small amounts of dietary cholesterol and phospholipids are also consumed. The three lipases responsible for lipid digestion are lingual lipase, gastric lipase, and pancreatic lipase. However, because the pancreas is the only consequential source of lipase, virtually all lipid digestion occurs in the small intestine. Pancreatic lipase breaks down each triglyceride into two free fatty acids and a monoglyceride. The fatty acids include both short-chain (less than 10 to 12 carbons) and long-chain fatty acids.
Nucleic acid digestion
The nucleic acids DNA and RNA are found in most of the foods you eat. Two types of pancreatic nuclease are responsible for their digestion: deoxyribonuclease, which digests DNA, and ribonuclease, which digests RNA. The nucleotides produced by this digestion are further broken down by two intestinal brush border enzymes (nucleosidase and phosphatase) into pentoses, phosphates, and nitrogenous bases, which can be absorbed through the alimentary canal wall.
The large food molecules that must be broken down into subunits are summarised in the table below.
Carbohydrate | Monosaccharides: glucose, galactose and fructose |
Triglycerides | Glycerol and free fatty acids |
Source | Substance |
Proteins | Single amino acids, dipeptides and tripeptides |
Nucleic acids | Pentose sugars, phosphate and nitrogenous bases |
Absorption
The mechanical and chemical digestive processes have one goal: to convert food into molecules small enough to be absorbed by the epithelial cells of the intestinal villi. The absorptive capacity of the alimentary canal is almost endless. Each day, the alimentary canal processes up to 10 litres of food, liquids, and GI secretions, yet less than one litre enters the large intestine. Almost all ingested food, 80 percent of electrolytes, and 90 percent of water are absorbed in the small intestine. Although the entire small intestine is involved in the absorption of water and lipids, most absorption of carbohydrates and proteins occurs in the jejunum. Notably, bile salts and vitamin B12 are absorbed in the terminal ileum. By the time chyme passes from the ileum into the large intestine, it is essentially indigestible food residue (mainly plant fibres like cellulose), some water, and millions of bacteria (Figure 7.22).

Absorption can occur through five mechanisms: (1) active transport, (2) passive diffusion, (3) facilitated diffusion, (4) cotransport (or secondary active transport), and (5) endocytosis. As you will recall from Chapter 5: Membrane Transport, active transport refers to the movement of a substance across a cell membrane going from an area of lower concentration to an area of higher concentration (up the concentration gradient). In this type of transport, proteins within the cell membrane act as pumps, using cellular energy (ATP) to move the substance. Passive diffusion refers to the movement of substances from an area of higher concentration to an area of lower concentration, while facilitated diffusion refers to the movement of substances from an area of higher to an area of lower concentration using a carrier protein in the cell membrane.
Co-transport (secondary active transport) uses the movement of one molecule through the membrane from higher to lower concentration to power the movement of another from lower to higher. This is an additional method of active transport in addition to the types we looked at in Chapter 5: Membrane Transport. We saw in that chapter that the sodium-potassium pump is continually pumping sodium ions out of the cell, against its concentration gradient, which uses ATP (energy). This process forms a concentration gradient of sodium ions – if they are able to, they will travel from the outside of the cell to the inside of the cell by passive transport (no ATP). Secondary active transport uses the kinetic energy of the sodium ions to bring other compounds, against their concentration gradient into the cell (Figure 7.23). Many amino acids, as well as glucose, enter a cell this way. It is called secondary because the movement is not using ATP directly, but indirectly by using the concentration gradient that was established using ATP. The example shown in Figure 7.23 shows the two molecules moving in to the cell (in the same direction). This is called a symporter. There is also a type of protein channel where the two molecules go in opposite directions, called an antiporter (Figure 7.24).


Finally, endocytosis is a transportation process in which the cell membrane engulfs material. It requires energy, generally in the form of ATP.
Because the cell’s plasma membrane is made up of hydrophobic phospholipids, water-soluble nutrients must use transport molecules embedded in the membrane to enter cells. Moreover, substances cannot pass between the epithelial cells of the intestinal mucosa because these cells are bound together by tight junctions. Thus, substances can only enter blood capillaries by passing through the apical surfaces of epithelial cells and into the interstitial fluid. Water-soluble nutrients enter the capillary blood in the villi and travel to the liver via the hepatic portal vein.
In contrast to the water-soluble nutrients, lipid-soluble nutrients can diffuse through the plasma membrane. Once inside the cell, they are packaged for transport via the base of the cell and then enter the lacteals of the villi to be transported by lymphatic vessels to the systemic circulation via the thoracic duct. The absorption of most nutrients through the mucosa of the intestinal villi requires active transport fueled by ATP. The routes of absorption for each food category are summarised in Table 7.3 below.
Food | Breakdown products | Absorption mechanism | Entry to bloodstream | Destination |
Carbohydrates | Glucose | Co-transport with sodium ions | Capillary blood in villi | Liver via hepatic portal vein |
Carbohydrates | Galactose | Co-transport with sodium ions | Capillary blood in villi | Liver via hepatic portal vein |
Carbohydrates | Fructose | Facilitated diffusion | Capillary blood in villi | Liver via hepatic portal vein |
Protein | Amino acids | Co-transport with sodium ions | Capillary blood in villi | Liver via hepatic portal vein |
Lipids | Long-chain fatty acids | Diffusion into intestinal cells, where they are combined with proteins to create chylomicrons | Lacteals of villi | Systemic circulation via lymph entering thoracic duct |
Lipids | Monoacylglycerides | Diffusion into intestinal cells, where they are combined with proteins to create chylomicrons | Lacteals of villi | Systemic circulation via lymph entering thoracic duct |
Lipids | Short-chain fatty acids | Simple diffusion | Capillary blood in villi | Liver via hepatic portal vein |
Lipids | Glycerol | Simple diffusion | Capillary blood in villi | Liver via hepatic portal vein |
Lipids | Nucleic acid digestion products | Active transport via membrane carriers | Capillary blood in villi | Liver via hepatic portal vein |
Carbohydrate absorption
All carbohydrates are absorbed in the form of monosaccharides. The small intestine is highly efficient at this, absorbing monosaccharides at an estimated rate of 120 grams per hour. All normally digested dietary carbohydrates are absorbed; indigestible fibres are eliminated in the faeces.
Protein absorption
Active transport mechanisms, primarily in the duodenum and jejunum, absorb most proteins as their breakdown products, amino acids. Almost all (95 to 98 percent) protein is digested and absorbed in the small intestine. The type of carrier that transports an amino acid varies.
Lipid absorption
About 95 percent of lipids are absorbed in the small intestine. Bile salts not only speed up lipid digestion, they are also essential to the absorption of the end products of lipid digestion. Short-chain fatty acids are relatively water soluble and can enter the absorptive cells (enterocytes) directly. Despite being hydrophobic, the small size of short-chain fatty acids enables them to be absorbed by enterocytes via simple diffusion, and then take the same path as monosaccharides and amino acids into the blood capillary of a villus.
The large and hydrophobic long-chain fatty acids and monoacylglycerides are not so easily suspended in the watery intestinal chyme. However, bile salts and lecithin resolve this issue by enclosing them in a micelle, which is a tiny sphere with polar (hydrophilic) ends facing the watery environment and hydrophobic tails turned to the interior. The core also includes cholesterol and fat-soluble vitamins. Without micelles, lipids would sit on the surface of chyme and never come in contact with the absorptive surfaces of the epithelial cells. Micelles can easily squeeze between microvilli and get very near the luminal cell surface. At this point, lipid substances exit the micelle and are absorbed via simple diffusion (Figure 7.25).

Nucleic acid absorption
The products of nucleic acid digestion—pentose sugars, nitrogenous bases, and phosphate ions—are transported by carriers across the villus epithelium via active transport. These products then enter the bloodstream.
Mineral absorption
The electrolytes absorbed by the small intestine are from both GI secretions and ingested foods. Since electrolytes dissociate into ions in water, most are absorbed via active transport throughout the entire small intestine. During absorption, there is an accumulation of sodium ions inside the cells, and a reduction of the potassium ion concentration inside the cells. To restore the sodium-potassium gradient across the cell membrane, a sodium-potassium pump requiring ATP pumps sodium out and potassium in.
In general, all minerals that enter the intestine are absorbed, whether you need them or not. Iron and calcium are exceptions; they are absorbed in the duodenum in amounts that meet the body’s current requirements, as follows:
Iron: The ionic iron needed for the production of haemoglobin is absorbed into mucosal cells via active transport. Once inside mucosal cells, ionic iron binds to the protein ferritin, creating iron-ferritin complexes that store iron until needed. When the body has enough iron, most of the stored iron is lost when worn-out epithelial cells slough off. When the body needs iron because, for example, it is lost during acute or chronic bleeding, there is increased uptake of iron from the intestine and accelerated release of iron into the bloodstream. Since women experience significant iron loss during menstruation, they have around four times as many iron transport proteins in their intestinal epithelial cells as do men.
Calcium: Blood levels of ionic calcium determine the absorption of dietary calcium. When blood levels of ionic calcium drop, parathyroid hormone (PTH) secreted by the parathyroid glands stimulates the release of calcium ions from bone matrices and increases the reabsorption of calcium by the kidneys. PTH also upregulates the activation of vitamin D in the kidney, which then facilitates intestinal calcium ion absorption.
Vitamin absorption
The small intestine absorbs the vitamins that occur naturally in food and supplements. Fat-soluble vitamins (A, D, E, and K) are absorbed along with dietary lipids in micelles via simple diffusion. This is why you are advised to eat some fatty foods when you take fat-soluble vitamin supplements. Most water-soluble vitamins (including most B vitamins and vitamin C) also are absorbed by simple diffusion. An exception is vitamin B12, which is a very large molecule. Intrinsic factor secreted in the stomach binds to vitamin B12, preventing its digestion and creating a complex that binds to mucosal receptors in the terminal ileum, where it is taken up by endocytosis.
Water absorption
Each day, about nine litres of fluid enter the small intestine. About 2.3 litres are ingested in foods and beverages, and the rest is from GI secretions. About 90 percent of this water is absorbed in the small intestine. Water absorption is driven by the concentration gradient of the water: The concentration of water is higher in chyme than it is in epithelial cells. Thus, water moves down its concentration gradient from the chyme into cells. As noted earlier, much of the remaining water is then absorbed in the colon.
acid a substance that donates hydrogen ions and therefore lowers pH
amino acid a monomer of a protein
base a substance that absorbs hydrogen ions and therefore raises pH
buffer a solution that resists a change in pH by absorbing or releasing hydrogen or hydroxide ions
carbohydrate a biological macromolecule in which the ratio of carbon to hydrogen to oxygen is 1:2:1; carbohydrates serve as energy sources and structural support in cells
cellulose a polysaccharide that makes up the cell walls of plants and provides structural support to the cell
chemical bond an interaction between two or more of the same or different elements that results in the formation of molecules
chitin a type of carbohydrate that forms the outer skeleton of arthropods, such as insects and crustaceans, and the cell walls of fungi
colon forms the major part of the large intestine
chyme the partially digested food and gastric juice mixture resulting from the mechanical and chemical digestion in the stomach
denaturation the loss of shape in a protein as a result of changes in temperature, pH, or exposure to chemicals
disaccharide two sugar monomers that are linked together by a peptide bond
enzyme a catalyst in a biochemical reaction that is usually a protein
fat a lipid molecule composed of three fatty acids and a glycerol (triglyceride) that typically exists in a solid form at room temperature
gastric relating to the stomach
glycogen a storage carbohydrate in animals
hormone a chemical signaling molecule, usually a protein or steroid, secreted by an endocrine gland or group of endocrine cells; acts to control or regulate specific physiological processes
hydrophilic describes a substance that dissolves in water; water-loving
hydrophobic describes a substance that does not dissolve in water; water-fearing
large intestine much smaller in length compared to the small intestine but larger in diameter, reabsorbs the water from indigestible food material and processes the waste material
lipids a class of macromolecules that are nonpolar and insoluble in water
oesophagus the long tube that connects the mouth to the stomach
oral cavity mouth
macromolecule a large molecule, often formed by polymerisation of smaller monomers
monosaccharide a single unit or monomer of carbohydrates
oil an unsaturated fat that is a liquid at room temperature
pepsin enzyme that digests protein
phospholipid a major constituent of the membranes of cells; composed of two fatty acids and a phosphate group attached to the glycerol backbone
polypeptide a long chain of amino acids linked by peptide bonds
polysaccharide a long chain of monosaccharides; may be branched or unbranched
protein a biological macromolecule composed of one or more chains of amino acids
rectum distal portion of the large intestine, stores faeces until defecation
salivary glands found in and around the mouth and produce and secrete saliva
saturated fatty acid a long-chain hydrocarbon with single covalent bonds in the carbon chain; the number of hydrogen atoms attached to the carbon skeleton is maximised
small intestine long tube-like organ where the digestion of food is completed, and is the site of nutrient absorption into the blood
sphincter a ring-shaped muscle that relaxes or tightens to open or close a passage or opening in the body
starch a storage carbohydrate in plants
steroid a type of lipid composed of four fused hydrocarbon rings
stomach saclike organ that secretes digestive juices
trans-fat a form of unsaturated fat with the hydrogen atoms neighbouring the double bond across from each other rather than on the same side of the double bond
triglyceride a fat molecule; consists of three fatty acids linked to a glycerol molecule
unsaturated fatty acid a long-chain hydrocarbon that has one or more than one double bonds in the hydrocarbon chain
Review Questions
Macromolecules
Chapter attribution
Content adapted from:
Anatomy and Physiology 2e (2022), by J. Gordon Betts, Kelly A. Young, James A. Wise, Eddie Johnson, Brandon Poe, Dean H. Kruse, Oksana Korol, Jody E. Johnson, Mark Womble and Peter DeSaix, is published by OpenStax https://openstax.org/details/books/anatomy-and-physiology-2e, and used under a CC BY licence.
Concepts of Biology (2013), by Samantha Fowler, Rebecca Roush and James Wise, is published by OpenStax https://openstax.org/details/books/concepts-biology, and used under a CC BY licence.
Microbiology (2016), by Nina Parker, Mark Schneegurt, Anh-Hue Thi Tu, Philip Lister and Brian M. Forster, is published by OpenStax https://openstax.org/details/books/microbiology, and used under a CC BY licence.
Media Attributions
- Figure 7.1 Food platter © Available under a PxHere licence
- Figure 7.2 Methane © Samantha Fowler, Rebecca Roush & James Wise is licensed under a CC BY (Attribution) license
- Figure 7.3 Macromolecules © Samantha Fowler, Rebecca Roush & James Wise is licensed under a CC BY (Attribution) license
- Figure 7.4 Examples of monosaccharides © Connie Rye, Robert Wise, Vladimir Jurukovski, Jean DeSaix, Jung Choi & Yael Avissar is licensed under a CC BY (Attribution) license
- Figure 7.5 Polysaccharide examples © Samantha Fowler, Rebecca Roush & James Wise is licensed under a CC BY (Attribution) license
- Figure 7.6 Otter © Michael Malz is licensed under a CC BY (Attribution) license
- Figure 7.7 Lipid examples © Samantha Fowler, Rebecca Roush & James Wise is licensed under a CC BY (Attribution) license
- Figure 7.8 Types of unsaturated fats © OpenStax
- Figure 7.9 Amino acids © Samantha Fowler, Rebecca Roush & James Wise is licensed under a CC BY (Attribution) license
- Figure 7.10 The four levels of protein structure © National Human Genone Research Institute adapted by Connie Rye, Robert Wise, Vladimir Jurukovski, Jean DeSaix, Jung Choi & Yael Avissar is licensed under a CC BY (Attribution) license
- Figure 7.11 The human digestion system © Connie Rye, Robert Wise, Vladimir Jurukovski, Jean DeSaix, Jung Choi & Yael Avissar is licensed under a CC BY (Attribution) license
- Figure 7.12 The mouth © Mariana Ruiz Villareal adapted by Charles Molnar & Jane Gair is licensed under a CC BY (Attribution) license
- Figure 7.13 Histology of the small intestine © J. Gordon Betts, Kelly A. Young, James A. Wise, Eddie Johnson, Brandon Poe, Dean H. Kruse, Oksana Korol, Jody E. Johnson, Mark Womble, & Peter DeSaix is licensed under a CC BY-NC-SA (Attribution NonCommercial ShareAlike) license
- Figure 7.14 Lower intestinal tract © J. Gordon Betts, Kelly A. Young, James A. Wise, Eddie Johnson, Brandon Poe, Dean H. Kruse, Oksana Korol, Jody E. Johnson, Mark Womble & Peter DeSaix is licensed under a CC BY (Attribution) license
- Figure 7.15 Large intestine © J. Gordon Betts, Kelly A. Young, James A. Wise, Eddie Johnson, Brandon Poe, Dean H. Kruse, Oksana Korol, Jody E. Johnson, Mark Womble & Peter DeSaix is licensed under a CC BY (Attribution) license
- Figure 7.16 Exocrine and endocrine pancreas © J. Gordon Betts, Kelly A. Young, James A. Wise, Eddie Johnson, Brandon Poe, Dean H. Kruse, Oksana Korol, Jody E. Johnson, Mark Womble & Peter DeSaix is licensed under a CC BY (Attribution) license
- Figure 7.17 Digestive processes © J. Gordon Betts, Kelly A. Young, James A. Wise, Eddie Johnson, Brandon Poe, Dean H. Kruse, Oksana Korol, Jody E. Johnson, Mark Womble, & Peter DeSaix is licensed under a CC BY (Attribution) license
- Figure 7.18 Digestion and Absorption © J. Gordon Betts, Kelly A. Young, James A. Wise, Eddie Johnson, Brandon Poe, Dean H. Kruse, Oksana Korol, Jody E. Johnson, Mark Womble & Peter DeSaix is licensed under a CC BY (Attribution) license
- Figure 7.19 Carbohydrate Digestion © J. Gordon Betts, Kelly A. Young, James A. Wise, Eddie Johnson, Brandon Poe, Dean H. Kruse, Oksana Korol, Jody E. Johnson, Mark Womble & Peter DeSaix is licensed under a CC BY (Attribution) license
- Figure 7.20 Protein Digestion © J. Gordon Betts, Kelly A. Young, James A. Wise, Eddie Johnson, Brandon Poe, Dean H. Kruse, Oksana Korol, Jody E. Johnson, Mark Womble & Peter DeSaix is licensed under a CC BY (Attribution) license
- Figure 7.21 Protein digestion flow chart © J. Gordon Betts, Kelly A. Young, James A. Wise, Eddie Johnson, Brandon Poe, Dean H. Kruse, Oksana Korol, Jody E. Johnson, Mark Womble, Peter DeSaix is licensed under a CC BY (Attribution) license
- Figure 7.22 Digestive secretions and absorption © J. Gordon Betts, Kelly A. Young, James A. Wise, Eddie Johnson, Brandon Poe, Dean H. Kruse, Oksana Korol, Jody E. Johnson, Mark Womble & Peter DeSaix is licensed under a CC BY (Attribution) license
- Figure 7.23 Electrochemical gradient © Mariana Ruiz Villarreal is licensed under a Public Domain license
- Figure 7.2 Uniporters, symporters and antiporters © Lupask is licensed under a Public Domain license
- Figure 7.25 Lipid absorption © J. Gordon Betts, Kelly A. Young, James A. Wise, Eddie Johnson, Brandon Poe, Dean H. Kruse, Oksana Korol, Jody E. Johnson, Mark Womble & Peter DeSaix is licensed under a CC BY (Attribution) license