5. Membrane transport

- Describe the molecular components that make up the cell membrane
- Describe the major features and properties of the cell membrane
- Differentiate between materials that can and cannot diffuse through the lipid bilayer
- Compare and contrast different types of passive transport with active transport, providing examples of each.
Despite differences in structure and function, all living cells in multicellular organisms have a surrounding cell membrane. As the outer layer of your skin separates your body from its environment, the cell membrane (also known as the plasma membrane) separates the inner contents of a cell from its exterior environment. This cell membrane provides a protective barrier around the cell and regulates which materials can pass in or out.
5.1 | How is the structure of the cell membrane related to its function?
The cell membrane is an extremely pliable structure composed primarily of back-to-back phospholipids (a bilayer). Cholesterol is also present, which contributes to the fluidity of the membrane, and there are various proteins embedded within the membrane that have a variety of functions.
How do phospholipids form a barrier between the inside and outside of the cell?
A single phospholipid molecule has a phosphate group on one end, called the head, and two side-by-side chains of fatty acids that make up the lipid tails (Figure 5.2a). The phosphate group is negatively charged, making the head polar and hydrophilic—or water loving. A hydrophilic molecule (or region of a molecule) is one that is attracted to water. The phosphate heads are thus attracted to the water molecules of both the extracellular and intracellular environments. The lipid tails, on the other hand, are uncharged, or non-polar, and are hydrophobic—or water fearing. A hydrophobic molecule (or region of a molecule) repels and is repelled by water. The tails are constantly in motion. If several phospholipid molecules are placed in water, they will self-assemble in a circle in a bilayer as shown in Figure 5.2b below. Soap is made up similar molecules that assemble in the same manner. Therefore, the hydrophilic portion can dissolve in water while the hydrophobic portion can trap grease in micelles (structures like in Figure 5.2b) that then can be washed away.
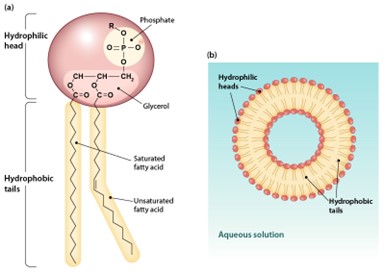
The important point to remember is that the phospholipid bilayer effectively establishes a barrier between internal and external environments.
The cell membrane consists of two adjacent layers of phospholipids. The lipid tails of one layer face the lipid tails of the other layer, meeting at the interface of the two layers. The phospholipid heads face outward, one layer exposed to the interior of the cell and one layer exposed to the exterior (Figure 5.3). Because the phosphate groups are polar and hydrophilic, they are attracted to water in the intracellular fluid. Intracellular fluid (ICF) is the fluid in the interior of the cell. The phosphate groups are also attracted to the extracellular fluid. Extracellular fluid (ECF) is the fluid environment outside the enclosure of the cell membrane. Because the lipid tails are hydrophobic, they meet in the inner region of the membrane, excluding watery intracellular and extracellular fluid from this space. The cell membrane has many proteins, as well as other lipids (such as cholesterol), that are associated with the phospholipid bilayer. An important feature of the membrane is that it remains fluid; the lipids and proteins in the cell membrane are not rigidly locked in place (Figure 5.4 and click on Figure 5.5 to see short movie).

How do proteins allow particles and messages through the membrane?
The lipid bilayer forms the basis of the cell membrane, but it is peppered throughout with various proteins. Two different types of proteins that are commonly associated with the cell membrane are the integral proteins and peripheral protein. As its name suggests, an integral protein is a protein that is embedded in the membrane (Figures 5.4 and 5.5).

Some types of integral proteins are:
- Channel protein which selectively allows particular materials, such as certain ions, to pass into or out of the cell.
- Cell recognition proteins (for example, glycoproteins), which serve to mark a cell’s identity so that it can be recognised by other cells.
- Receptor proteins that can selectively bind a specific molecule outside the cell, and this binding induces a chemical reaction within the cell.
Peripheral proteins are typically found on the inner or outer surface of the lipid bilayer but can also be attached to the internal or external surface of an integral protein. These proteins typically perform a specific function for the cell. Some peripheral proteins on the surface of intestinal cells, for example, act as digestive enzymes (enzymes are proteins!) to break down nutrients to sizes that can pass through the cells and into the bloodstream. The plasma membrane is fluid, not rigid (See video below:[ 1:27])
5.2 | How can substances move across the cell membrane?
One of the great wonders of the cell membrane is its ability to regulate the concentration of substances inside the cell. These substances include ions such as Ca++, Na+, K+, and Cl–; nutrients including sugars, fatty acids, and amino acids; and waste products, particularly carbon dioxide (CO2), which must leave the cell.
The membrane’s lipid bilayer structure provides the first level of control. The phospholipids are tightly packed together, and the membrane has a hydrophobic interior. This structure causes the membrane to be selectively permeable. A membrane that has selective permeability allows only substances meeting certain criteria to pass through it unaided. In the case of the cell membrane, only relatively small, non-polar materials can move through the lipid bilayer (remember, the lipid tails of the membrane are non-polar). Some examples of these are other lipids, oxygen and carbon dioxide gases, and alcohol. However, water-soluble materials—like glucose, amino acids, and electrolytes—need some assistance to cross the membrane because they are repelled by the hydrophobic tails of the phospholipid bilayer. All substances that move through the membrane do so by one of two general methods, which are categorised based on whether or not energy is required.
- Passive transport is the movement of substances across the membrane without the expenditure of cellular energy.
- Active transport, in contrast, is the movement of substances across the membrane using energy; this energy comes from the molecule adenosine triphosphate (ATP).
Passive transport
In order to understand how substances move passively across a cell membrane, it is necessary to understand concentration gradients and diffusion. A concentration gradient is the difference in concentration of a substance across a space. Molecules (or ions) will spread/diffuse from where they are more concentrated to where they are less concentrated until they are equally distributed in that space. When molecules move in this way, they are said to move down their concentration gradient. Diffusion is the movement of particles from an area of higher concentration to an area of lower concentration. A couple of common examples will help to illustrate this concept. Imagine being inside a closed bathroom. If a bottle of perfume were sprayed, the scent molecules would naturally diffuse from the spot where the perfume was sprayed to all corners of the bathroom, and this diffusion would go on until no more concentration gradient remained. Another example is a spoonful of sugar placed in a cup of tea. Eventually, the sugar will diffuse throughout the tea until no concentration gradient remains. In both cases, if the room is warmer or the tea hotter, diffusion occurs even faster as the molecules are bumping into each other and spreading out faster than at cooler temperatures. Having an internal body temperature around 37.5oC thus also aids in diffusion of particles within the body.
Whenever a substance exists in greater concentration on one side of a semipermeable membrane, such as the cell membrane, any substance that can move down its concentration gradient across the membrane will do so. Consider substances that can easily diffuse through the lipid bilayer of the cell membrane, such as the gases oxygen (O2) and carbon dioxide (CO2).
Because cells rapidly use up oxygen during metabolism, there is typically a lower concentration of O2 inside the cell than outside. As a result, oxygen will diffuse from outside the cell through the lipid bilayer of the membrane and into the cytoplasm within the cell. On the other hand, because cells produce CO2 as a by-product of metabolism, CO2 concentrations rise within the cell; therefore, CO2 will move from the cell through the lipid bilayer to outside the cell, where its concentration is lower. This mechanism of molecules spreading from where they are more concentrated to where they are less concentration is a form of passive transport called simple diffusion (Figure 5.5). O2 generally diffuses into cells because it is more concentrated outside of them, and CO2 typically diffuses out of cells because it is more concentrated inside of them. Neither of these examples requires any energy on the part of the cell, and therefore they are said to use passive transport to move across the membrane.

Solutes dissolved in water on either side of the cell membrane will tend to diffuse down their concentration gradients, but because most substances cannot pass freely through the lipid bilayer of the cell membrane, their movement is restricted as they can only pass through protein channels and specialised transport mechanisms in the membrane (Figure 5.6). Facilitated diffusion is the diffusion process used for those substances that cannot cross the lipid bilayer due to their size and/or polarity. A common example of facilitated diffusion is the movement of glucose into the cell, where it is used to make ATP. Although glucose can be more concentrated outside of a cell, it cannot cross the lipid bilayer via simple diffusion because it is both large and polar. To resolve this, a specialised carrier protein called the glucose transporter will transfer glucose molecules into the cell to facilitate its inward diffusion.

Another example is sodium ions (Na+) which are highly concentrated outside of cells; however, these ions are polarised and cannot pass through the non-polar lipid bilayer of the membrane. Their diffusion across the cell membrane is facilitated by membrane proteins that form sodium channels (or “pores”), so that Na+ ions can move down their concentration gradient from outside the cells to inside the cells. There are many other solutes that must undergo facilitated diffusion to move into a cell, such as amino acids, or to move out of a cell, such as wastes. Because facilitated diffusion is a passive process, it does not require energy expenditure by the cell.
Water also can move freely across the cell membrane of all cells, either through protein channels or by slipping between the lipid tails of the membrane itself. Osmosis is the diffusion of water through a semipermeable membrane (Figure 5.7).

The movement of water molecules is not itself regulated by cells, so it is important that cells are exposed to an environment in which the concentration of solutes outside of the cells (in the extracellular fluid) is equal to the concentration of solutes inside the cells (in the cytoplasm). Two solutions that have the same concentration of solutes are said to be isotonic (equal tension). When cells and their extracellular environments are isotonic, the concentration of water molecules is the same outside and inside the cells, and the cells maintain their normal shape (and function).
Osmosis occurs when there is an imbalance of solutes outside of a cell versus inside the cell. A solution that has a higher concentration of solutes than another solution is said to be hypertonic, and water molecules tend to diffuse into a hypertonic solution. In contrast, a solution that has a lower concentration of solutes than another solution is said to be hypotonic, and water molecules tend to diffuse out of a hypotonic solutions into hypertonic solutions, as illustrated in the example shown in Figure 5.7.
Figure 5.8 illustrates what happens to red blood cells when they are placed in solutions of different tonicity. Cells in a hypertonic solution will shrivel as water leaves the cell via osmosis. Cells in a hypotonic solution will take on too much water and swell, with the risk of eventually bursting. Lastly, cells in an isotonic solution remain unchanged. Therefore, it makes sense that the fluid (plasma) that red blood cells float around in, is isotonic. A critical aspect of homeostasis in living things is to create an internal environment in which all of the body’s cells are in an isotonic solution. Various organ systems, particularly the kidneys, work to maintain this homeostasis.
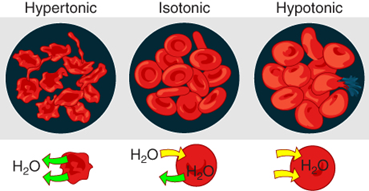
Another mechanism besides diffusion to passively transport materials between compartments is filtration. Unlike diffusion of a substance from where it is more concentrated to less concentrated, filtration uses a hydrostatic pressure gradient that pushes the fluid—and the solutes within it—from a higher pressure area to a lower pressure area. Filtration is an extremely important process in the body. For example, the circulatory system uses filtration to move plasma and substances across the endothelial (the inside of capillaries is lined with endothelial cells) lining of capillaries and into surrounding tissues, supplying cells with the nutrients. Filtration pressure in the kidneys provides the mechanism to remove wastes from the bloodstream.
Active transport
For all of the transport methods described above, the cell expends no energy. Membrane proteins that aid in the passive transport of substances do so without the use of ATP. In contrast, during active transport, ATP is required to move a substance across a membrane, often with the help of protein carriers, and usually against the concentration gradient of the substance.
We have discussed simple concentration gradients—differential concentrations of a substance across a space or a membrane—but in living systems, gradients are more complex. Because cells contain proteins, most of which are negatively charged, and because ions move into and out of cells, there is an electrical gradient, a difference of charge, across the plasma membrane. The interior of living cells is electrically negative with respect to the extracellular fluid in which they are bathed; at the same time, cells have higher concentrations of potassium ions (K+) and lower concentrations of sodium ions (Na+) than does the extracellular fluid. Thus, in a living cell, the concentration gradient and electrical gradient of Na+ promotes diffusion of the ion into the cell, and the electrical gradient of Na+ (a positive ion) tends to drive it inward to the negatively charged interior. The situation is more complex, however, for other elements such as potassium. The electrical gradient of K+ promotes diffusion of the ion into the cell, but the concentration gradient of K+ promotes diffusion out of the cell (Figure 5.9). The combined gradient that affects an ion is called its electrochemical gradient, and it is especially important to muscle and nerve cells.

Moving against a gradient
To move substances against a concentration or an electrochemical gradient, the cell must use energy. This energy is harvested from ATP that is generated through cellular metabolism. Active transport mechanisms, collectively called pumps or carrier proteins, work against electrochemical gradients. With the exception of ions, small substances constantly pass through plasma membranes. Active transport maintains concentrations of ions and other substances needed by living cells in the face of these passive changes. Much of a cell’s supply of metabolic energy may be spent maintaining these processes. Because active transport mechanisms depend on cellular metabolism for energy, they are sensitive to many metabolic poisons that interfere with the supply of ATP.
Two mechanisms for active transport exist for the transport of small-molecular weight material and macromolecules. Primary active transport moves ions across a membrane and creates a difference in charge across that membrane. The primary active transport system uses ATP to move a substance, such as an ion, into the cell, and often at the same time, a second substance is moved out of the cell. The sodium-potassium pump, an important pump in animal cells, expends energy to move potassium ions into the cell and a different number of sodium ions out of the cell (Figure 5.10). The action of this pump results in a concentration and charge difference across the membrane.
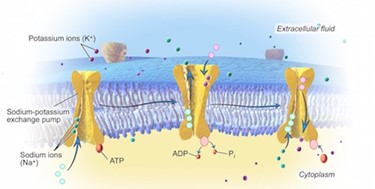
In addition to moving small ions and molecules through the membrane, cells also need to remove and take in larger molecules and particles. Some cells are even capable of engulfing entire unicellular microorganisms. You might have correctly hypothesised that the uptake and release of large particles by the cell requires energy. A large particle, however, cannot pass through the membrane, even with energy supplied by the cell; specialised processes exist for this.
Endocytosis is a type of active transport that moves particles, such as large molecules, parts of cells, and even whole cells, into a cell. There are different variations of endocytosis, but all share a common characteristic: The plasma membrane of the cell invaginates, forming a pocket around the target particle. The pocket pinches off, resulting in the particle being contained in a newly created vacuole that is formed from the plasma membrane. Below, we discuss three types of endocytosis (Figure 5.11).
Phagocytosis is the process by which large particles, such as cells, are taken in by a cell. For example, when microorganisms invade the human body, a type of white blood cell called a neutrophil removes the invader through this process, surrounding and engulfing the microorganism (Figure 5.11a), which is then destroyed by the neutrophil . A variation of endocytosis is called pinocytosis. This literally means “cell drinking” and was named at a time when the assumption was that the cell was purposefully taking in extracellular fluid. In reality, this process takes in solutes that the cell needs from the extracellular fluid (Figure 5.11b).

A targeted variation of endocytosis employs binding proteins in the plasma membrane that are specific for certain substances (Figure 5.11c). The particles bind to the receptors (proteins on the external plasma membrane surface) and the plasma membrane invaginates, bringing the substance and the receptors into the cell. If passage across the membrane of the target of receptor-mediated endocytosis is ineffective, it will not be removed from the tissue fluids or blood. Instead, it will stay in those fluids and increase in concentration. Some human diseases are caused by a failure of receptor-mediated endocytosis. For example, the form of cholesterol termed low-density lipoprotein or LDL (also referred to as bad cholesterol) is removed from the blood by receptor-mediated endocytosis. In the human genetic disease familial hypercholesterolemia, the LDL receptors are defective or missing entirely. People with this condition have life-threatening levels of cholesterol in their blood, because their cells cannot clear the chemical from their blood.
In contrast to these methods of moving material into a cell is the process of exocytosis. Exocytosis is the opposite of the processes discussed above in that its purpose is to expel material from the cell into the extracellular fluid. A particle enveloped in membrane (for example, a vesicle) fuses with the interior of the plasma membrane. This fusion opens the membranous envelope to the exterior of the cell, and the particle is expelled into the extracellular space (Figure 5.12). Hormones and neurotransmitters (chemicals released by neuronal cells) are released by exocytosis.

5.3 | How does the cell membrane affect movement between the body’s fluid compartments?
In the previous chapter, we looked at the properties of water that are important for the human body and introduced the different fluid compartments within the body: the intracellular fluid; the extracellular fluid and the interstitial fluid. The chemical reactions of life take place in the aqueous solutions of these fluids. The dissolved substances in a solution are called solutes. In the human body, solutes vary in different parts of the body, but may include proteins, lipids, carbohydrates, and, very importantly, electrolytes. In Chapter 3: Chemistry for Life and Chapter 4: Water Biology we noted that in medicine, a mineral dissociated from a salt that carries an electrical charge (an ion) is called an electrolyte. For instance, sodium ions (Na+) and chloride ions (Cl-) are often referred to as electrolytes.
Now we have seen that in the body, water moves through semi-permeable membranes of cells and from one compartment of the body to another by a process called osmosis. Osmosis is basically the diffusion of water from regions of higher concentration to regions of lower concentration, along an osmotic gradient across a semi-permeable membrane. As a result, water will move into and out of cells and tissues, depending on the relative concentrations of the water and solutes found there. An appropriate balance of solutes inside and outside of cells must be maintained to ensure normal function.
chemical gradient refers to the concentration of an ion or a molecule
concentration gradient occurs when a substance is more concentrated in one area than another
diffusion the passive movement of molecules or particles along a concentration gradient
electrical gradient a difference of charge across the cell membrane
electrochemical gradient combination of chemical gradient and electrical gradient
endocytosis type of active transport that moves particles or even whole cells into a cell
extracellular fluid all the fluid that is outside of cells
exocytosis active process (requiring ATP) that expels material from a cell
facilitated diffusion form of passive transport across a biological membrane in which a transporter protein facilitates the movement of an otherwise membrane-impermeable molecule or ion across the plasma membrane down its concentration gradient
hydrophilic water-loving
hydrophobic water-hating
hypertonic solution contains a higher concentration of solute compared to another solution
hypotonic solution contains a lower concentration of solute compared to another solution
intercellular fluid located between cells
intracellular fluid located within the cell
isotonic solution contains the same concentration of solute compared to another
metabolic energy energy derived from chemical processes in the cell
metabolism the sum of chemical reactions that take place within each cell of a living organism
selective permeability property of a membrane that allows only some substances to pass across it unaided
tonicity a description of the relative solute concentration in a solution as compared to another solution
Review Questions
Chapter attribution
Content adapted from:
Anatomy and Physiology 2e (2022), by J. Gordon Betts, Kelly A. Young, James A. Wise, Eddie Johnson, Brandon Poe, Dean H. Kruse, Oksana Korol, Jody E. Johnson, Mark Womble and Peter DeSaix, is published by OpenStax https://openstax.org/details/books/anatomy-and-physiology-2e, and used under a CC BY licence.
Concepts of Biology (2013), by Samantha Fowler, Rebecca Roush and James Wise, is published by OpenStax https://openstax.org/details/books/concepts-biology, and used under a CC BY licence.
Microbiology (2016), by Nina Parker, Mark Schneegurt, Anh-Hue Thi Tu, Philip Lister and Brian M. Forster, is published by OpenStax https://openstax.org/details/books/microbiology, and used under a CC BY licence.
Media Attributions
- Figure 5.1 Bilipid layers of adjacent cells © Shigeki Watanabe & Erik Jorgensen, used with permission, is licensed under a All Rights Reserved license
- Figure 5.2 A phospholipid molecule © OpenStax is licensed under a CC BY (Attribution) license
- Figure 5.3 Phospholipid bilayer © J. Gordon Betts, Kelly A. Young, James A. Wise, Eddie Johnson, Brandon Poe, Dean H. Kruse, Oksana Korol, Jody E. Johnson, Mark Womble & Peter DeSaix is licensed under a CC BY (Attribution) license
- Figure 5.4 Cell membrane. © J. Gordon Betts, Kelly A. Young, James A. Wise, Eddie Johnson, Brandon Poe, Dean H. Kruse, Oksana Korol, Jody E. Johnson, Mark Womble & Peter DeSaix is licensed under a CC BY (Attribution) license
- Figure 5.6 Simple diffusion across the cell membrane. © J. Gordon Betts, Kelly A. Young, James A. Wise, Eddie Johnson, Brandon Poe, Dean H. Kruse, Oksana Korol, Jody E. Johnson, Mark Womble & Peter DeSaix is licensed under a CC BY (Attribution) license
- Figure 5.7 Facilitated diffusion. © J. Gordon Betts, Kelly A. Young, James A. Wise, Eddie Johnson, Brandon Poe, Dean H. Kruse, Oksana Korol, Jody E. Johnson, Mark Womble & Peter DeSaix is licensed under a CC BY (Attribution) license
- Figure 5.8 Osmosis is the diffusion of water through a semipermeable membrane down its concentration gradient © J. Gordon Betts, Kelly A. Young, James A. Wise, Eddie Johnson, Brandon Poe, Dean H. Kruse, Oksana Korol, Jody E. Johnson, Mark Womble, Peter DeSaix is licensed under a CC BY (Attribution) license
- Figure 5.9 Water movement from cells in different solutions © J. Gordon Betts, Kelly A. Young, James A. Wise, Eddie Johnson, Brandon Poe, Dean H. Kruse, Oksana Korol, Jody E. Johnson, Mark Womble & Peter DeSaix is licensed under a CC BY (Attribution) license
- Figure 5.10 Electrochemical gradients © Synaptidude adapted by Charles Molnar & Jane Gair is licensed under a CC BY (Attribution) license
- Figure 5.11 Sodium potassium exchange pump © BruceBlaus is licensed under a CC BY (Attribution) license
- Figure 5.12 Endocytosis © Mariana Ruiz Villarreal LadyofHats adapted by Samantha Fowler, Rebecca Roush & James Wise is licensed under a Public Domain license
- Figure 5.13 Exocytosis © J. Gordon Betts, Kelly A. Young, James A. Wise, Eddie Johnson, Brandon Poe, Dean H. Kruse, Oksana Korol, Jody E. Johnson, Mark Womble & Peter DeSaix is licensed under a CC BY (Attribution) license